Spektr-RG/SRG
Non-EO
Astronomy and Telescopes
Quick facts
Overview
Mission type | Non-EO |
Spektr-RG / SRG (Spectrum Roentgen Gamma) astrophysical observatorySpacecraft Launch Mission Status Sensor Complement References
Spektr-RG/SRG is an international cooperative space research and technology demonstration mission of Roskosmos (Russia), ESA (Europen Space Agency), IKI (Space Research Institute), Moscow; MPE (Max-Planck-Institute for extraterrestrial Physics), Garching, Germany, and NASA/MSFC, Huntsville, AL, USA. A MOU (Memorandum of Understanding) was signed in March 2007 between DLR and Roskosmos. On Aug. 18, 2009, DLR and Roskosmos signed a detailed agreement during the MAKS International Aviation and Space Salon in Moscow, specifying all the organizational and technical boundary conditions for the eROSITA project. 1) 2)
The overall objective of the mission is to conduct the first all-sky survey with an imaging telescope in the 0.5-11 keV band to discover the hidden population of several hundred thousand obscured supermassive black holes and the first all-sky imaging X-ray time variability survey. In addition to the all-sky surveys it is foreseen to observe dedicated sky regions with high sensitivity to detect ten thousands of clusters of galaxies and thereafter to do follow-up pointed observations of selected sources, in order to investigate the nature of Dark Matter and Dark Energy. The proposed orbit provides an order of magnitude lower particle background than those of Chandra and XMM-Newton, which will allow the detailed study of low-surface-brightness diffuse objects.
The newly defined SRG mission represents a highly significant scientific and technological step beyond Chandra/XMM-Newton and is expected to provide important and timely inputs for the next generation of giant X-ray observatories like IXO (International X-ray Observatory) collaborative mission of ESA along with NASA and JAXA for the timeframe 2021.
The following arrangements were defined for the partners of the mission in Sept. 2005: 3) 4) 5)
• Roskosmos is the provider of the spacecraft bus and the launch of the satellite
• The eROSITA instrument is being developed by MPE Garching 6) 7)
• The ART-XC instrument and gamma-ray burst detector are being provided by RosKosmos (an IKI-led consortium)
• ESA will provide the communication subsystem and ground station support.
Background:
Initially (early 1990s), the high-energy international mission was called SXG (Spectrum-X Gamma), planned to be developed under the auspices of the Russian Space Agency, with instruments contributed by research groups in a number of European countries and the US. The payload consisted of a number of imaging instruments for cosmic photon observations in the energy range of 0.03 - 100 keV. A launch was planned for 1999. - However, due to repeated delays of the SXG project, caused by the economic situation in post-Soviet Russia, a new approach was taken in the timeframe 2004.
In the early SRG definition phase of the mission (2005-2007), there was a third payload in planning for SRG, namely the LWFT (Lobster-Eye Wide Field Telescope), a new technology introduction designed and developed by the Space Research Center of the University of Leicester, UK. However, the LWFT payload was removed from the SRG mission in 2008 due to budgetary problems in the UK.
The LWFT instrument is an all-sky X-ray monitor comprised of 5 telescope modules, each consisting of approximately 60 MCP (Microchannel Plate) optics, tiled to produce the required field of view and geometrical area. Each telescope module has a so-called “microwell array proportional counter” detector in the focal plane. 8) 9) 10) 11)
The ‘lobster-eye’ geometry which permits this huge sky coverage as well as high sensitivity, is achieved with square-pore MCPs based on the eyes of the crustaceans themselves (Figure 1) with Micromegas detectors. This configuration rotates to cover the whole sky in ~90 minutes in continuous slew mode with a given source transiting the FOV in 300-1800 s.
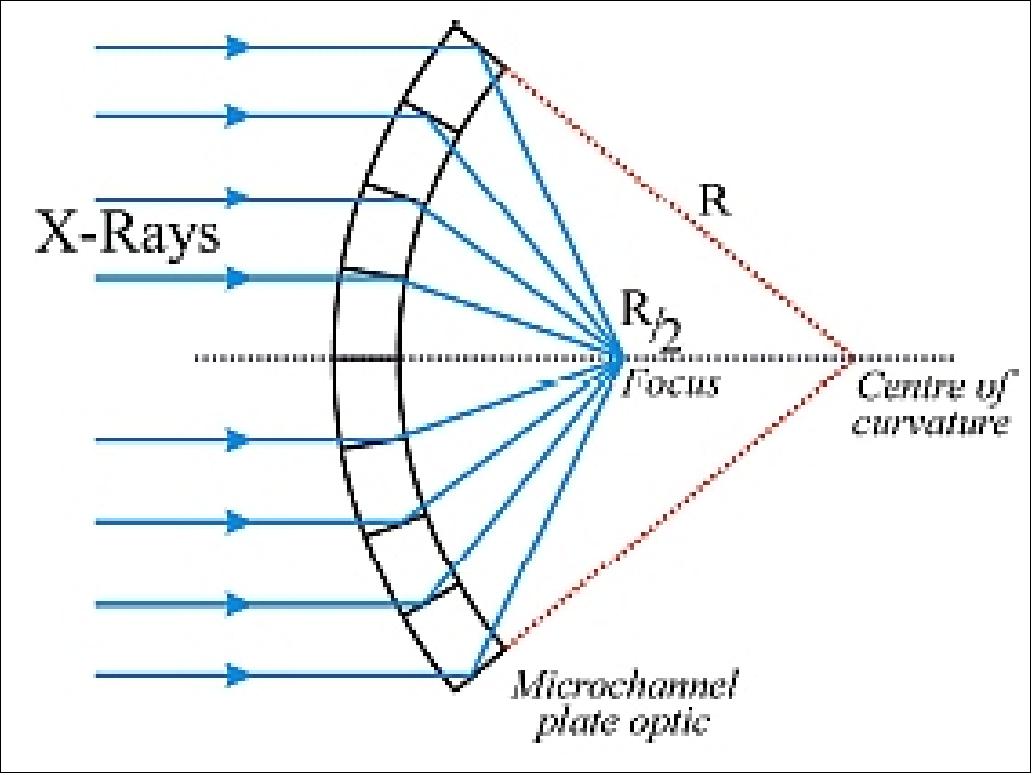
Spacecraft:
Spektr-RG (SRG) is an astrophysical space observatory, aimed at studying our Universe in the X-ray band of the electromagnetic spectrum once it is in position at the Lagrange point L2 of the Sun-Earth system, 1.5 million kilometers away from Earth.
The Spektr-RG astrophysical space observatory is developed by the Russian side under the Federal Space Program of Russia, section on Fundamental Space Research, by order of the Russian Academy of Sciences with the participation of Germany.
The SGR spacecraft is based on the 'Navigator' multi-use bus of the NPO Lavochkin Scientific Production Association, Khimki, Russia. The spacecraft is 3-axis stabilized and has an estimated launch mass of ~ 2650 kg (total payload mass of 1160 kg). 12) 13) 14) 15)
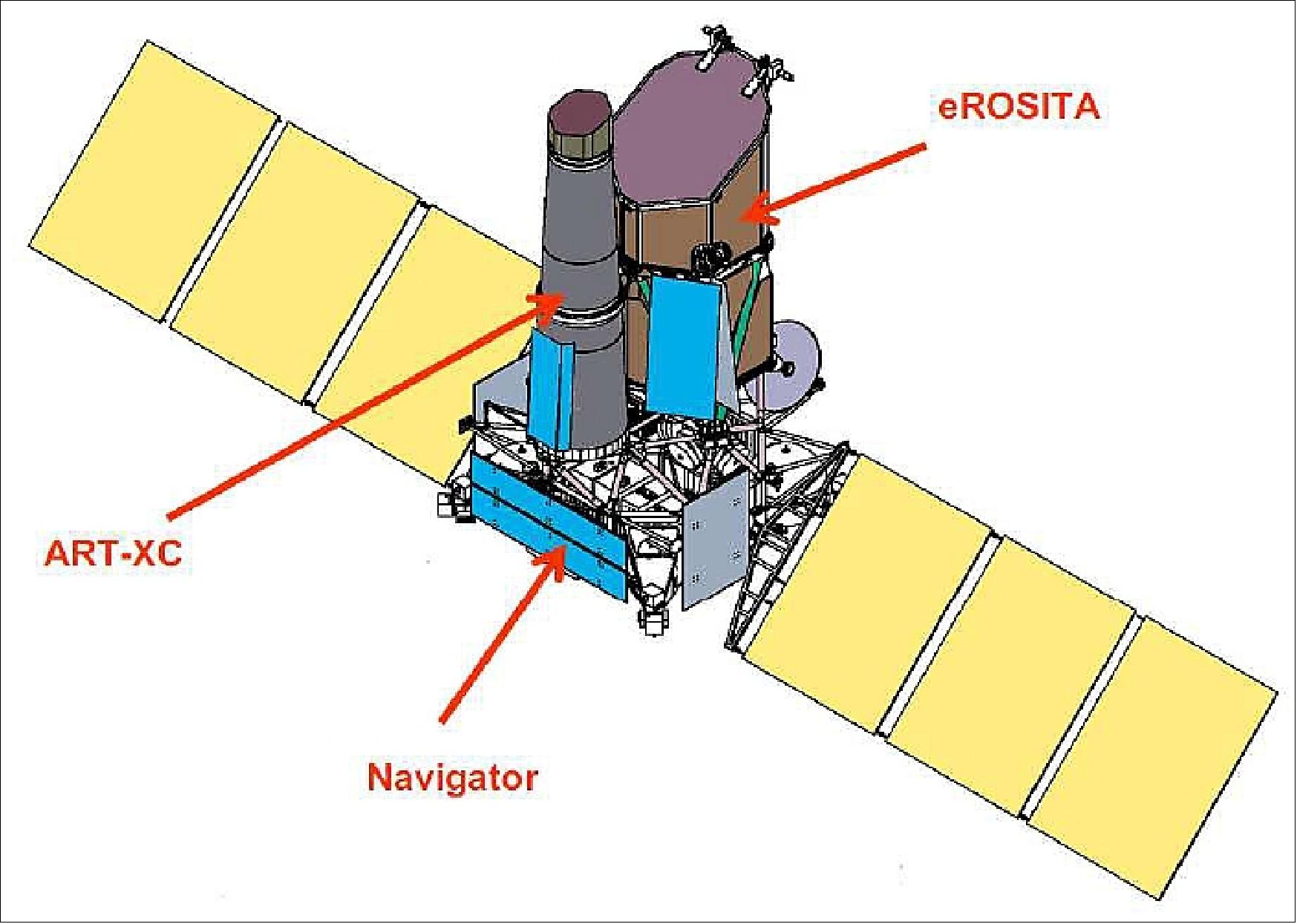
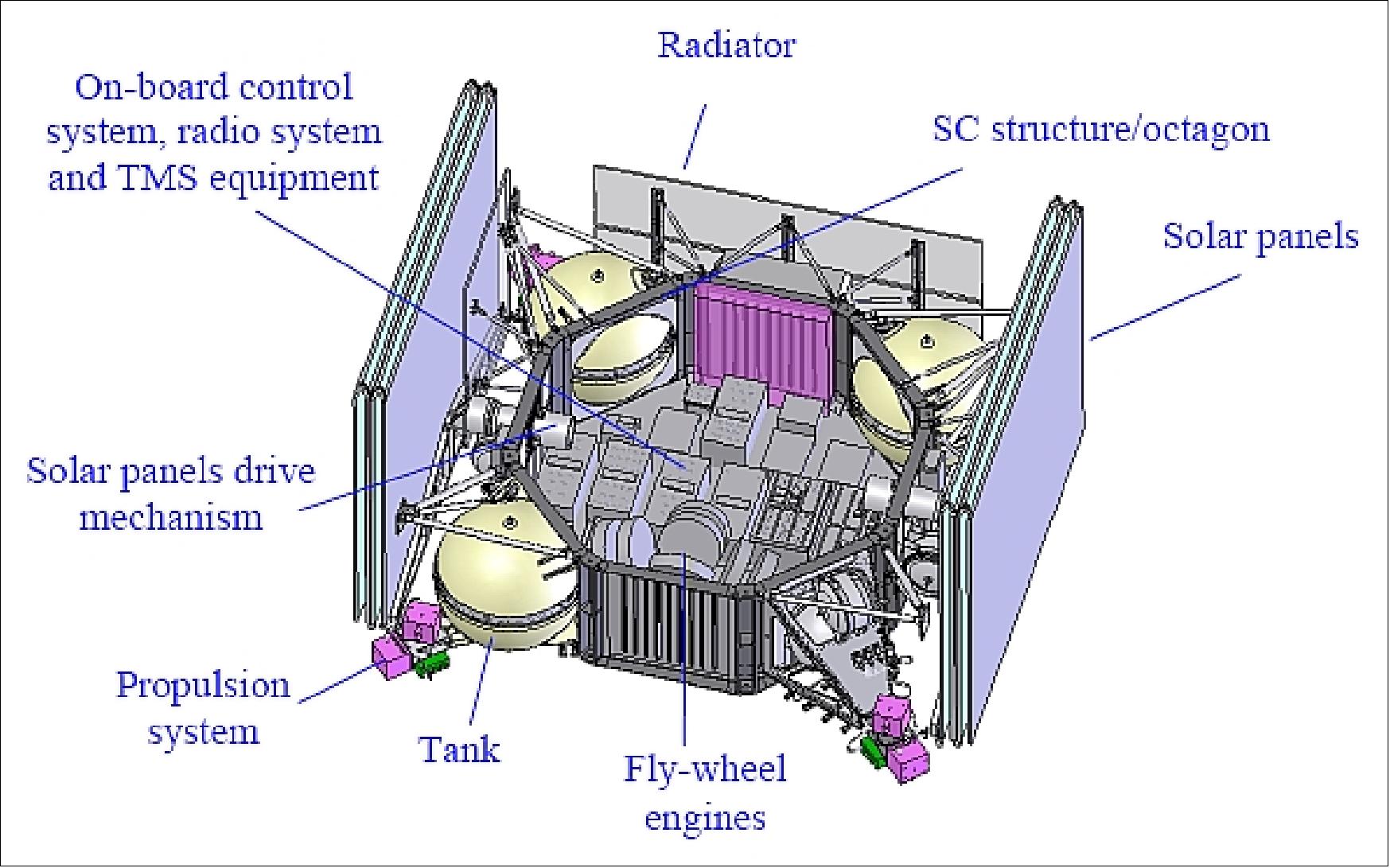
Spacecraft dry mass | 2350 kg, (wet mass: 2712.5 kg) |
Propellant (Hydrazine, helium) | Up to 300 kg |
Navigation and stabilization parameters | Pointing: 2 arcmin |
Power supply parameters | Supply voltage: 27 ± 1.35 V |
Design life | > 5 years (goal of 7.5 years) |
RF communications: Data transmission rate of 2 Mbit/s.
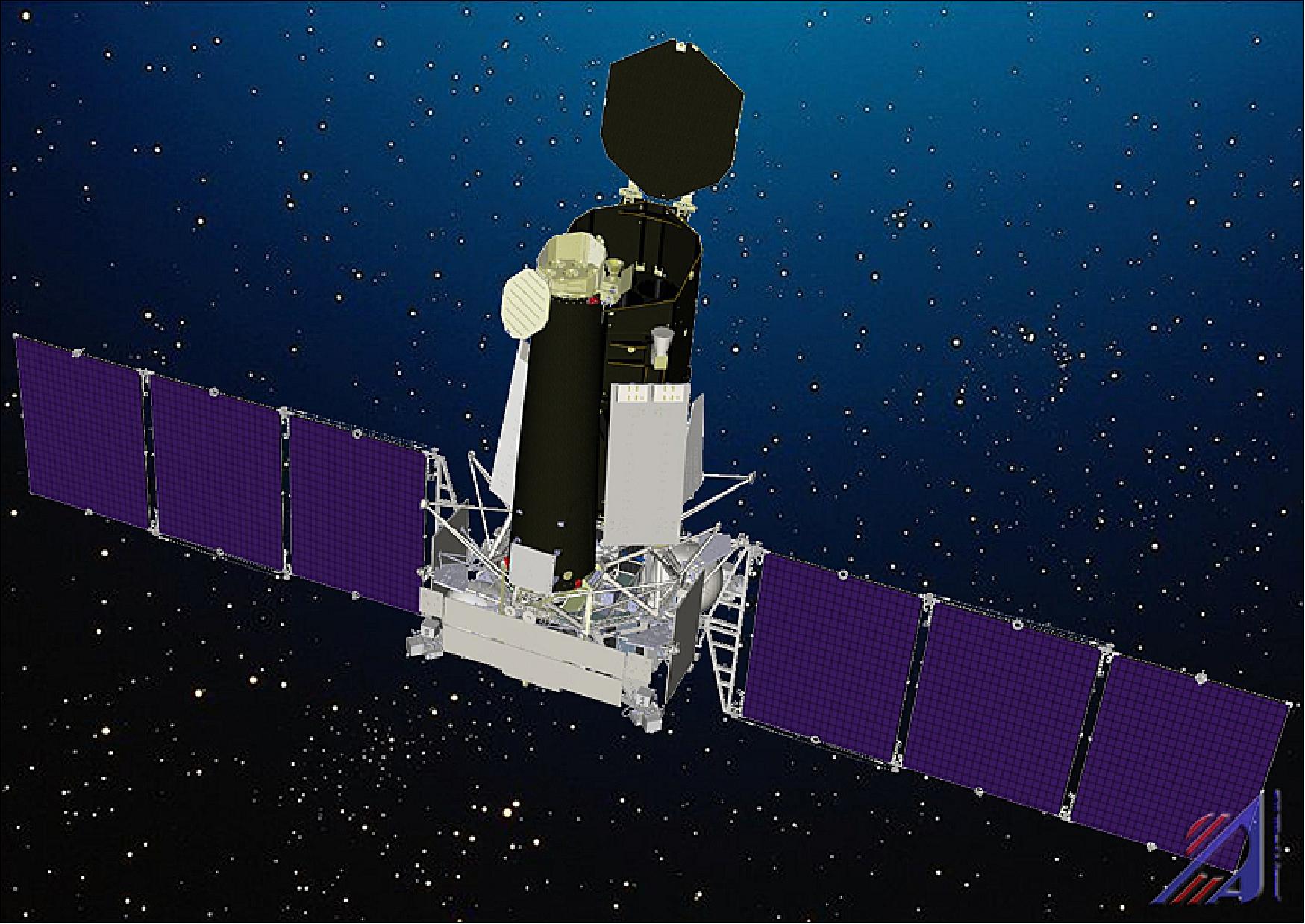
Development status:
• June 22, 2019: eROSITA marks the beginning of a new era in X-ray astronomy. Because no telescope before has ever focused on the entire sky in as much detail as eROSITA will. “We built eROSITA to transform the way we see the X-ray sky, and to unravel the mysteries of cosmology and black holes”, says Peter Predehl from the Max Planck Institute for Extraterrestrial Physics, the scientific Director of the mission. "The unprecedented spectral and spatial resolution will allow us to study the distribution of huge galaxy clusters and find out more about the mysterious dark energy”. 16)
- The question of the nature of the mysterious dark energy, which is tearing the universe apart at an accelerated rate, has occupied astronomers for many years. Dark energy accounts for almost 70% of the total mass of the universe. It eludes direct observation. But together with dark matter, which accounts for about 30% of space, it influences the formation and evolution of galaxy clusters; these are the largest gravitationally bound objects in the universe.
- The question of the nature of the mysterious dark energy, which is tearing the universe apart at an accelerated rate, has occupied astronomers for many years. Dark energy accounts for almost 70% of the total mass of the universe. It eludes direct observation. But together with dark matter, which accounts for about 30% of space, it influences the formation and evolution of galaxy clusters; these are the largest gravitationally bound objects in the universe.
- In a detailed sky survey, eROSITA will map the large-scale structure of the universe and observe around 100,000 galaxy clusters. The researchers are not only focussing their attention on the hot intergalactic medium in these clusters but also on gas and dust in between. On a large scale, these threads of matter give the cosmos the structure of a network; the galaxy clusters arrange themselves at the nodes of this network.
- The scientists also expect the X-ray telescope to detect millions of active galactic nuclei containing massive black holes. “Earlier this year, we saw the first image of a supermassive black hole sitting in the center of a galaxy. eROSITA will now tell us when and where this monster and a million others like it grew over cosmic time," states Kirpal Nandra, Director of the High-Energy Astrophysics group at MPE. "It’s staggering to think how much our understanding of the Universe has advanced."
- Within our Milky Way, eROSITA will also discover many X-ray sources, including double stars and the remains of stellar explosions (supernovae). Rare objects such as isolated neutron stars (i.e. the burnt out and super dense relics of dead, massive suns) are also on the observation plan.
• On 25 April 2019, the Spektr-RG Space Observatory was transported to the Baikonur Cosmodrome - the final stage in preparation for its launch on 21 June. Spektr-RG carries the X-ray telescope eROSITA, which was developed and built at MPE. Its aim is to perform a highly sensitive survey of the entire sky in X-ray light, once it arrives at its observation point L2. 17)
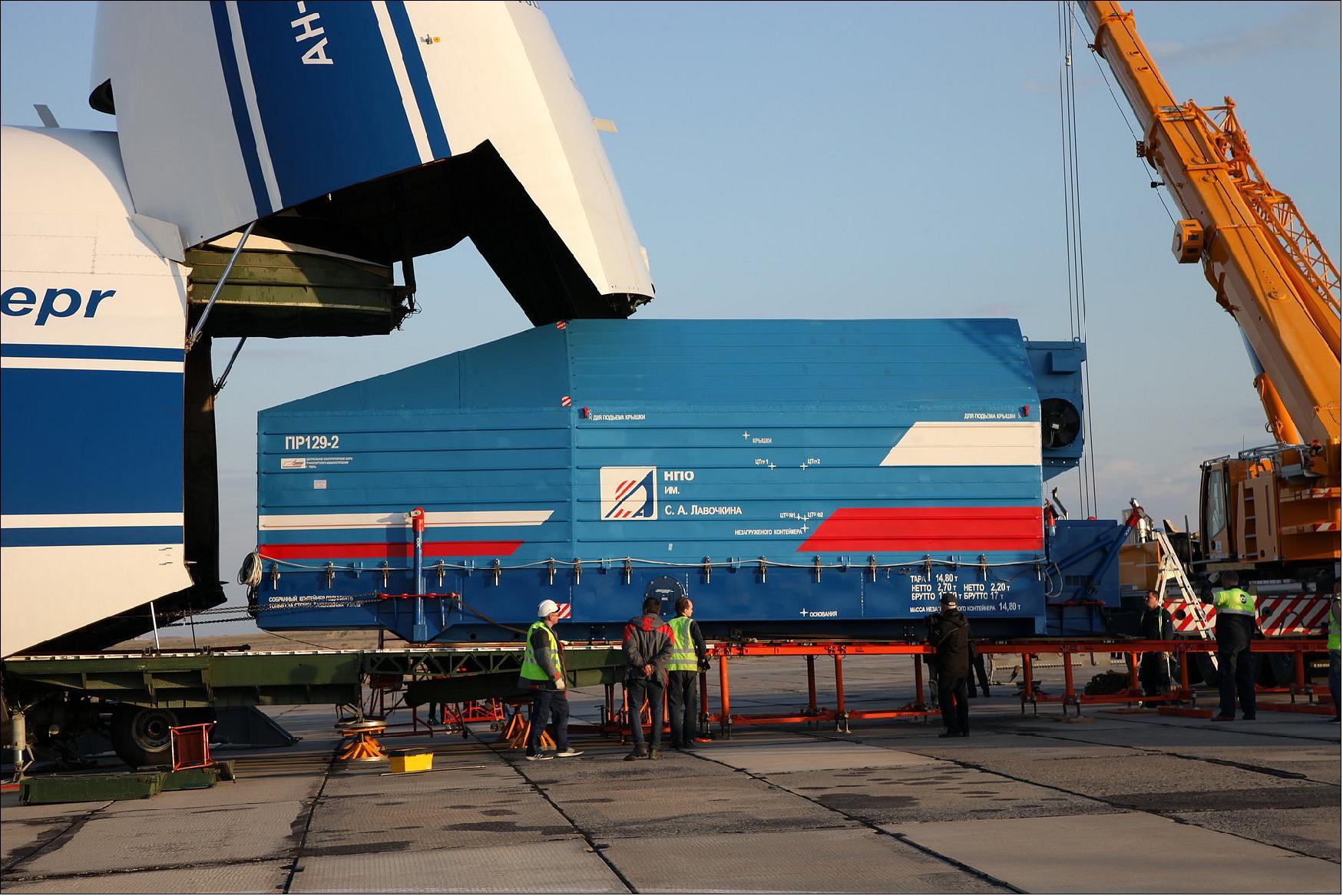
- In Baikonur, the Spektr-RG space observatory will be subject to final ground tests and will be integrated into the launch vehicle. The launch is planned for 21 June 2019 with a Proton M launcher and a DM-03 upper stage. Members of the eROSITA team will carry out final tests and preparations for launch at the beginning of May.
- The eROSITA X-ray space telescope consists of seven identical mirror modules with 54 nested gold-coated mirrors each, manufactured very precisely to collect the high-energy photons and guide them to the X-ray sensitive cameras placed at their focus. These cameras have also been developed and custom-built at MPE, containing special X-ray CCDs manufactured from high-purity silicon. For maximum performance, these cameras have to be cooled to -90°C using a complex heat pipe system.
- In addition to eROSITA, Spektr-RG also carries the Russian telescope “ART-XC”. Both instruments will fly from the Russian launch site Baikonur in Kazakhstan with a rocket to the second Lagrange point (L2) of the Sun-Earth system.
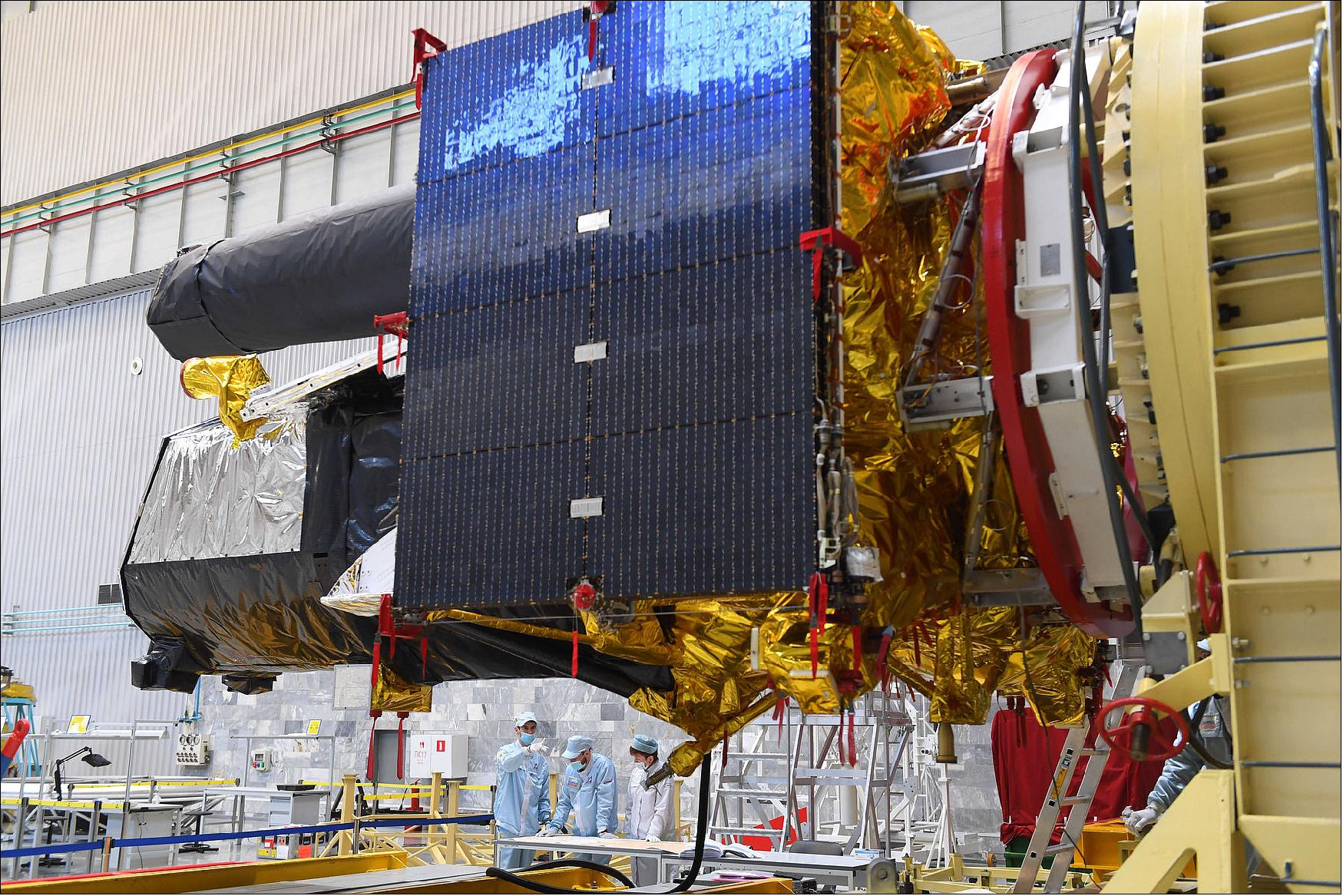
- Leaving Earth behind, eROSITA will then travel to the second Lagrange point (L2) of the Sun-Earth system, at a distance of about 1.5 million kilometers. Over a period of four years, eROSITA will carry out a total of eight scans of the entire sky to measure about 100,000 galaxy clusters, find about three million supermassive black holes and make many other interesting discoveries. This new sky survey, with much improved energy resolution and 20 times higher sensitivity than the previous one performed by ROSAT, will give scientists a closer insight into the origin and evolution of the universe.
• September 18, 2018: During 2018, Russian specialists conducted assembly and testing of the Spektr-RG X-ray observatory in preparation for launch in the following year. 18)
- On 19 April 2018, the press-service of the RKS Corporation said that it had delivered the Spektr-RG's radio system, BRK, and that the launch of the space observatory would occur in March 2019.
- By the end of May, both operational instruments of the observatory — the Russian ART-XC and the German eRosita — had been integrated with the flight version of the Navigator service module and tested. At the time, engineers were still working on a software update in the flight control system, BKU, of the spacecraft for handling the interface with the ART-XC instrument, industry sources said.
- On May 29, NPO Lavochkin announced that from May 3 to May 21, a joint team of its engineers and specialists from the Space Research Institute, IKI, in Moscow and Max Planck Institute in Germany had conducted electric tests of the flight-worthy onboard radio complex, BRK, integrated with eRosita and ART-XC telescopes. According to NPO Lavochkin, the electric tests were necessary to check interaction between the service systems of the spacecraft and scientific instruments. The company also said that the spacecraft had been transferred to the assembly room and was a process of preparation for thermal and vacuum tests which had been scheduled to begin in July.
- In the meantime, on May 22, Roskosmos abruptly cancelled a routine status meeting of participants in the Spektr-RG project scheduled in Moscow just two days later on May 24. According to the official explanation sent to the German side, a letter from the German space agency, DLR, requesting the review had come too late for the Russian side to process for the given date. However, the Russian leadership of the Spektr-RG project unofficially informed their German colleagues that the cancellation might be related to another management shakeup at the top of Roskosmos.
- The sources familiar with the situation expressed optimism that the Spektr-RG had remained on track for its latest launch window at the end of March — beginning of April 2019. At the time, the launch window for Spektr-RG was reported to be extending from February 27 to April 11, 2019.
• On January 20, 2017, the completed eROSITA X-ray telescope boarded a cargo plane and was transported from Munich, where it had been built at the MPE (Max Planck Institute for Extraterrestrial Physics), to Moscow. At NPO Lavochkin Scientific Production Association, Khimki near Moscow, eROSITA will be further tested and integrated with the SRG (Spectrum Roentgen Gamma) spacecraft in preparation for launch in spring 2018. 19)
- “With its much higher sensitivity than previous survey missions, eROSITA will discover a multitude of new X-ray sources,” expects Dr. Andrea Merloni, eROSITA project scientist. “We will be able to study not only the distribution of clusters of galaxies – eROSITA will detect more than 100,000 of these most massive bound objects in the Universe – but also millions of active black holes at the centers of galaxies, as well as rare objects in the Milky Way, such as isolated neutron stars. The survey will thus provide new insights into a wide range of high-energy astrophysical phenomena – maybe even reveal some completely new phenomena – and give us new clues about the mysterious “Dark Energy”, the force behind the accelerated expansion of the Universe.”
- SRG also carries the Russian telescope “ART-XC”. Both instruments will be launched with a Proton rocket from the Russian launch site Baikonur in Kazakhstan after another 2600 km transport.
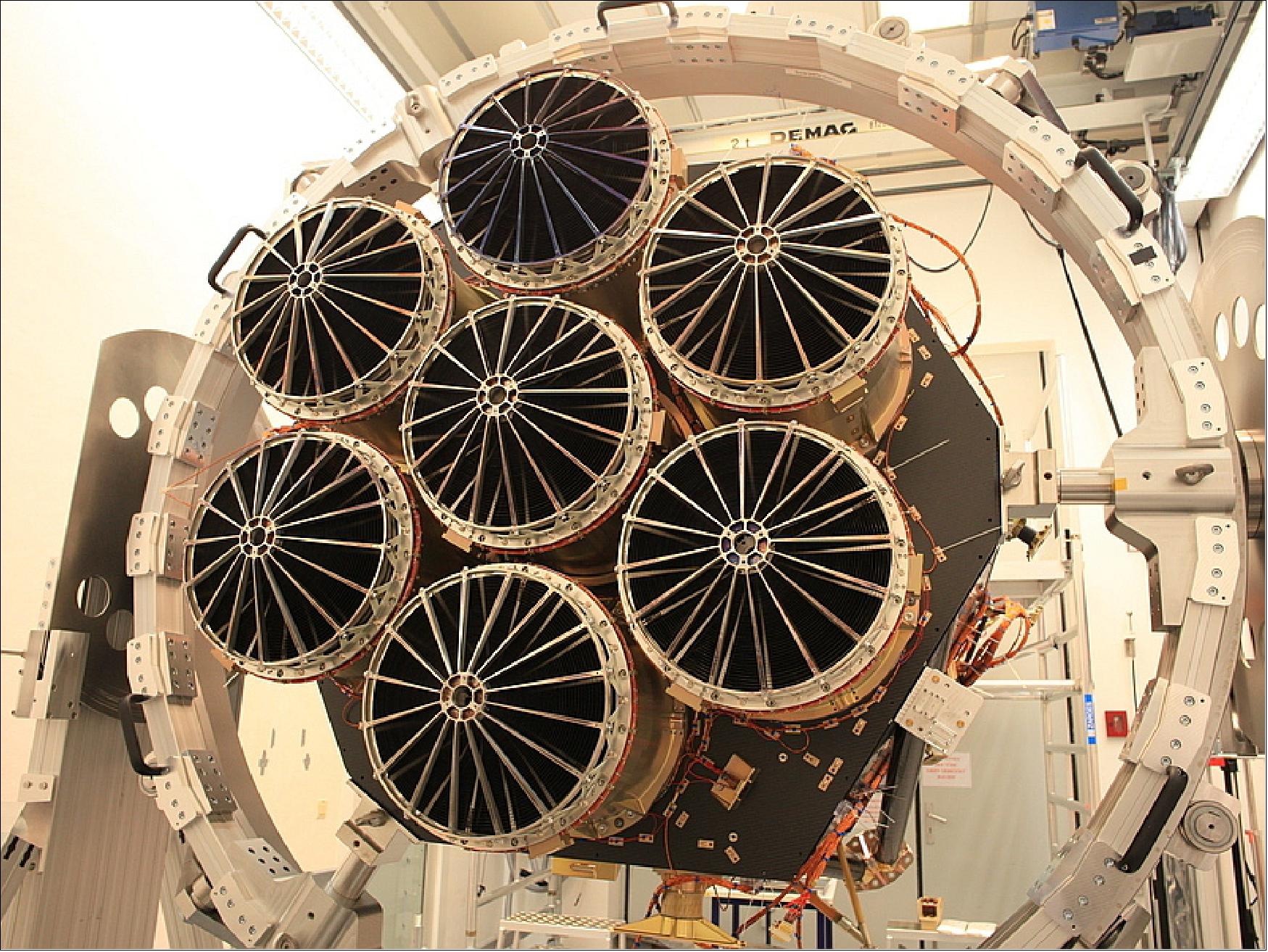
• December 2016: The Russian-built ART-XC telescope and the Navigator platform (which would carry both instruments into space), were reported to be in a high degree of readiness for flight. The ART-AC instrument was finally delivered from RKTs Progress in Samara to NPO Lavochkin by Dec. 27, 2016, according to Anatoly Zak.
- The final assembly and the integrated testing of the Spektr-RG / SRG spacecraft is expected to take around nine months, making the spacecraft available for the delivery to Baikonur around October 2017.
• December 2016: The final test campaign on the full telescope (vibration, mass properties and EMC) took place at IAGB (Ottobrunn), prior to the Moscow flight of eROSITA.
• November 2016: The eROSITA FM (Flight Model) telescope has been fully integrated in the MPE integration Lab. After completion of the work in September 2016, the telescope left MPE to be transported to the PANTER test facility, where it underwent an extensive test campaign. 20)
• April 2016: The final calibration of eROSITA's 8 MAs (Mirror Assemblies) is still ongoing in the PANTER facility of MPE, while the calibration of all CAs (Camera Assemblies) is underway in the smaller PUMA facility at MPE. 21)
- In parallel, the project started the preparation for the complete telescope integration (Figure 8), each of the 7 MA-CA pairs will be mounted first, thereby precisely adjusting the distance between mirror and camera to the individual focal lengths which has been measured during the mirror calibration.
- The SRG spacecraft is assembled in proto-flight configuration, waiting for the integration of the radio complex FM, expected for May 2016. The development for the GCS (Ground Control Segment) is ongoing, with no delays. The SRG GCC (Ground Control Center) will be operative in Q2 of 2017. A compatibility test with the MPE GCS is scheduled for early 2017. The launch vehicle are available and the SRG launch is confirmed for September 2017.
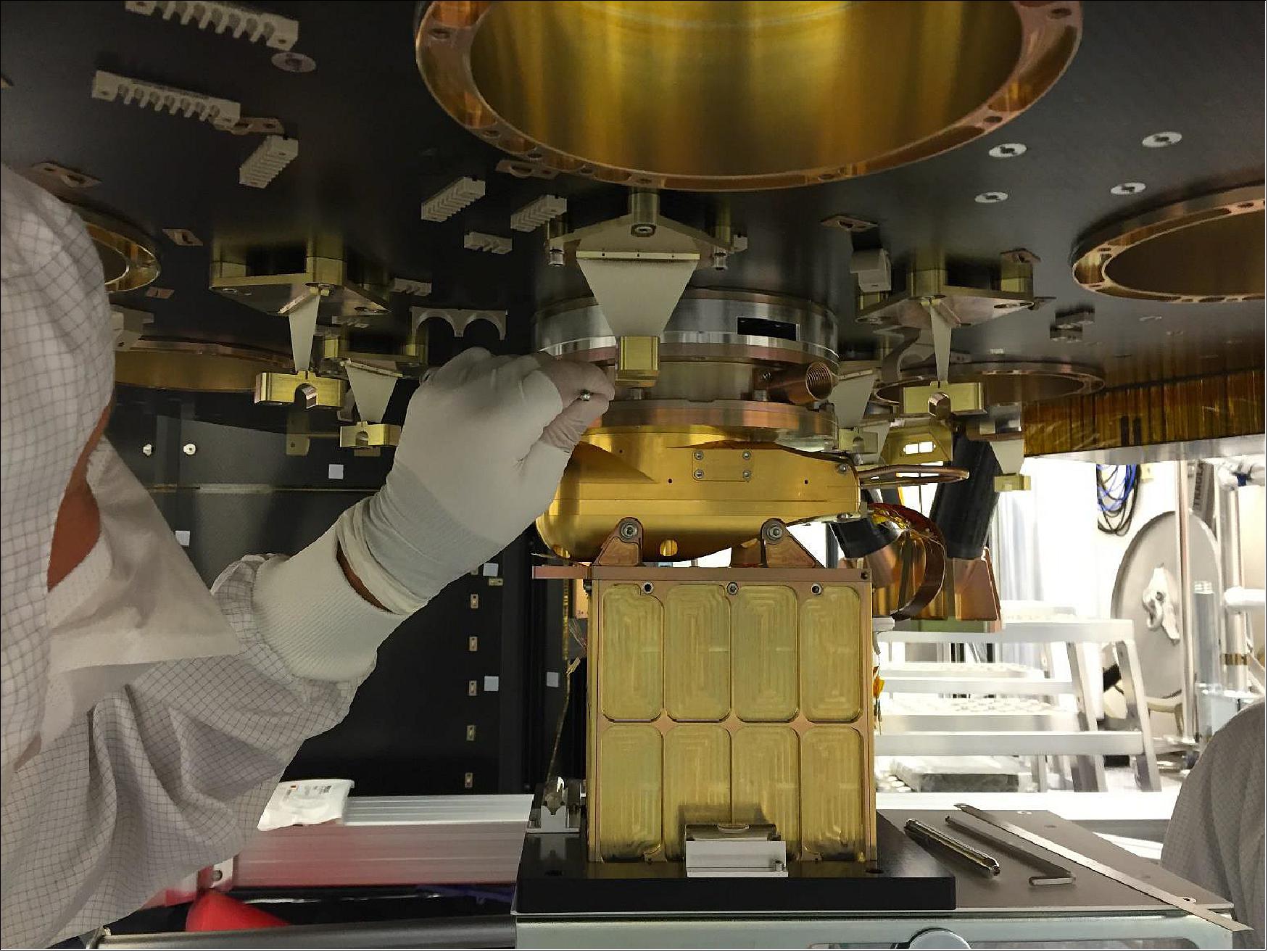
Launch: The Spektr-RG/SRG spacecraft (liftoff mass of 2,704 kg) was launched on 13 July 2019 (12:30:57 UTC) on a Proton/Block-DM-03 upper stage from the Baikonur Cosmodrome, Kazakhstan. 22) 23) 24) 25)
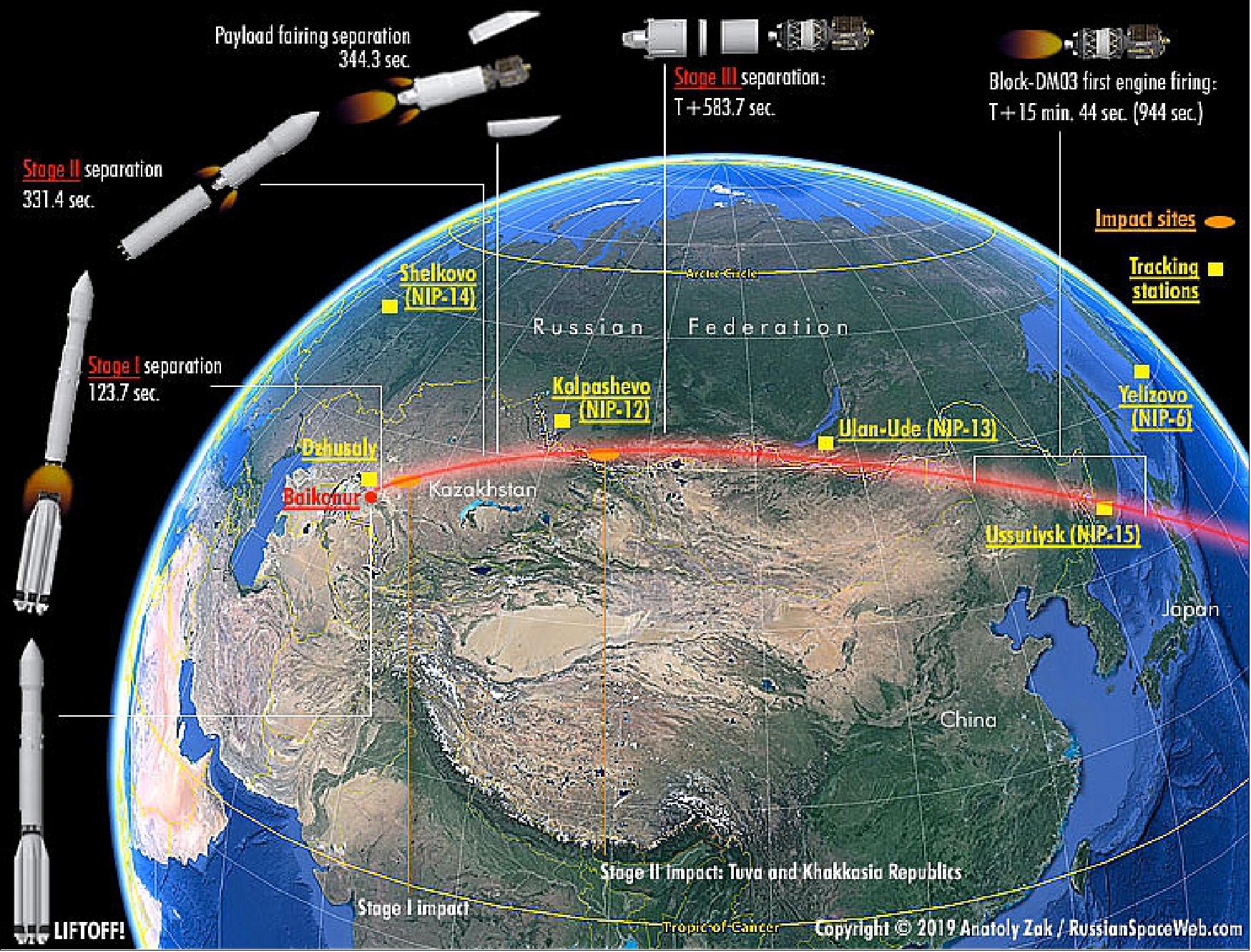
Orbit: The orbit of SRG has been selected to be at L2 (Lagrangian Point 2). The spacecraft will be in a Lissajous (or halo) orbit about the Lagrangian point L2. In the Sun‐Earth system the L2 point is on the rotating Sun-Earth axis about the same distance away as L1 (1.5 million km, representing 1/100 the distance from Earth to the Sun) but at the opposite side of the Earth. The L1 location is inside the Earth orbit while the L2 location is outside the Earth orbit. 26)
Note: The initial LEO circular orbit of the mission of 580 km altitude was revised in 2008 to a Lagrangian orbit at L2.
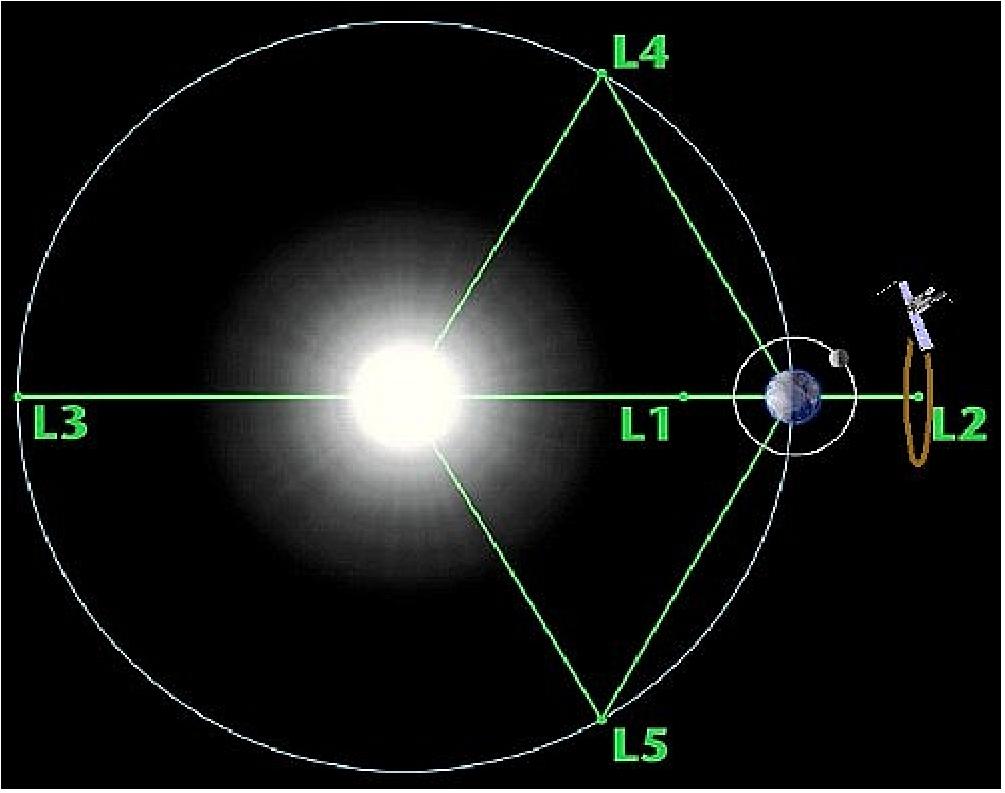
Observing strategy:
• The 3 months flight to L2 will be used in the verification and calibration of the payload.
• 4 years - duration of an all sky survey; 8 all sky surveys (scanning mode: 6 rotations/day, 1 degree advance per day)
• 3 years - follow-up period (goal) used for pointed observations of a selection of the most interesting galaxy clusters and AGNs (Active Galactic Nuclei).
Mission status
• March 2, 2022: Following the recommendation to freeze co-operation with Russia, eROSITA was placed into safe mode during the ground contact on Saturday 26th February. Science operations with the instrument are currently paused. SRG/eROSITA has now completed four of its intended eight all-sky survey passes. We hope that circumstances will permit a return to normal operations as soon as possible. Analysis of the existing German eROSITA data by the consortium led by MPE continues. 27)
• July 5, 2021: At today’s 16th Marcel Grossmann meeting, Dr. Peter Predehl accepted the Institutional Marcel Grossmann Award, which has been awarded to the Max Planck Institute for Extraterrestrial Physics (MPE). The institute receives the award jointly with the S.A. Lavochkin Association and the Space Research Institute (IKI) of the Russian Academy of Sciences “for the creation of the world’s best X-ray map of the entire sky”. 28)
- On behalf of MPE, Dr. Peter Predehl has been presented with the institutional Marcel Grossmann award “for the creation of the world's best X-ray map of the entire sky, for the discovery of millions of previously unknown accreting supermassive black holes at cosmological redshifts, for the detection of X-rays from tens of thousands of galaxy clusters, filled mainly with dark matter, and for permitting the detailed investigation of the growth of the large-scale structure of the universe during the era of dark energy dominance”. MPE shares the award with the S.A. Lavochkin Association and the Space Research Institute (IKI) of the Russian Academy of Sciences.
- MPE has received the award for the development of the eROSITA X-ray telescope aboard the Russian-German Spektr-RG mission, which was successfully launched from Baikonur on July 13, 2019 and placed in a halo orbit around the L2 point. It has since performed an all-sky X-ray survey with unprecedented sensitivity, spectral and angular resolution. The previous best map of this kind was provided by ROSAT, also an MPE-led project, more than 30 years ago. Already the first eROSITA map reached about 4 times deeper.
- “If anything, eROSITA and SRG are performing even better than we had hoped and expected,” said Predehl. “It’s a dream come true.”
- eROSITA was designed to detect at least 100.000 clusters of galaxies and map out the evolution of the large-scale structure of the Universe. The sky survey is also in the process of revealing millions of accreting supermassive black holes across cosmic time, and providing new insight into the physics of X-ray source populations in our galaxy, such as pre-main sequence stars, supernova remnants and X-ray binaries.
- Clusters of galaxies are the largest collapsed objects in the Universe and their formation and evolution is governed by the competition between gravity, i.e. Dark Matter, and the mysterious “Dark Energy” which is causing the expansion of the University to accelerate. X-ray observations of clusters of galaxies can be used to measure accurately key cosmological parameters such as the rate of expansion of the Universe and its evolution, the amount of dark matter, and the amplitude of primordial fluctuations, which are the origin of the whole structure of the universe.
- The eROSITA telescope consists of seven identical Wolter-1 mirror modules. Its novel detector system was developed by MPE based on the successful XMM-Newton pn-CCD technology. MPE is the scientific lead institute of eROSITA, responsible for the development of the instrument, the operation, the analysis software and data archive. In 2020, eROSITA completed the first of eight surveys and, at this time, already discovered more than 1 million X-ray sources, more than all X-ray observatories of the last 50 years together. This demonstrates that the design goals of the mission will easily be fulfilled.
- The award comes hot on the heels of the first public release of eROSITA data, together with a slew of scientific papers prepared by the German eROSITA collaboration that prove the discovery power of the instrument. “Under Peter Predehl’s leadership the eROSITA team has conceived, designed and built a state-of-the-art instrument. We are now successfully operating it in space, processing and analysing the data on the ground, and delivering on its scientific promise”, said Kirpal Nandra, Director at MPE and head of the High Energy Group. “It’s a remarkable achievement for the Institute and a delight for this to be recognized with this award, along with our partners in Russia.”
- As Principal Investigator, Predehl led the development of eROSITA and was German lead scientist of the SRG mission for more than a decade. But he is the first to point out that eROSITA’s success is the culmination of the efforts of an exceptionally talented, hard-working and dedicated team. „Many individual MPE members have received prizes in the past, but this is the first one for the institute as a whole”, he adds. “It’s great that the whole team effort has been recognized, and I am proud to have contributed to this amazing project.”
- The Marcel Grossmann Award is named for a Swiss mathematician and a friend and classmate of Albert Einstein. Since 1985, the International Center for Relativistic Astrophysics in Rome presents the awards for research in gravitation and astrophysics at the Marcel Grossmann meetings, which take place every three years. There are both individual and institutional awards. Each recipient receives a silver casting of the T. E. S. T. sculpture by the artist A. Pierelli.
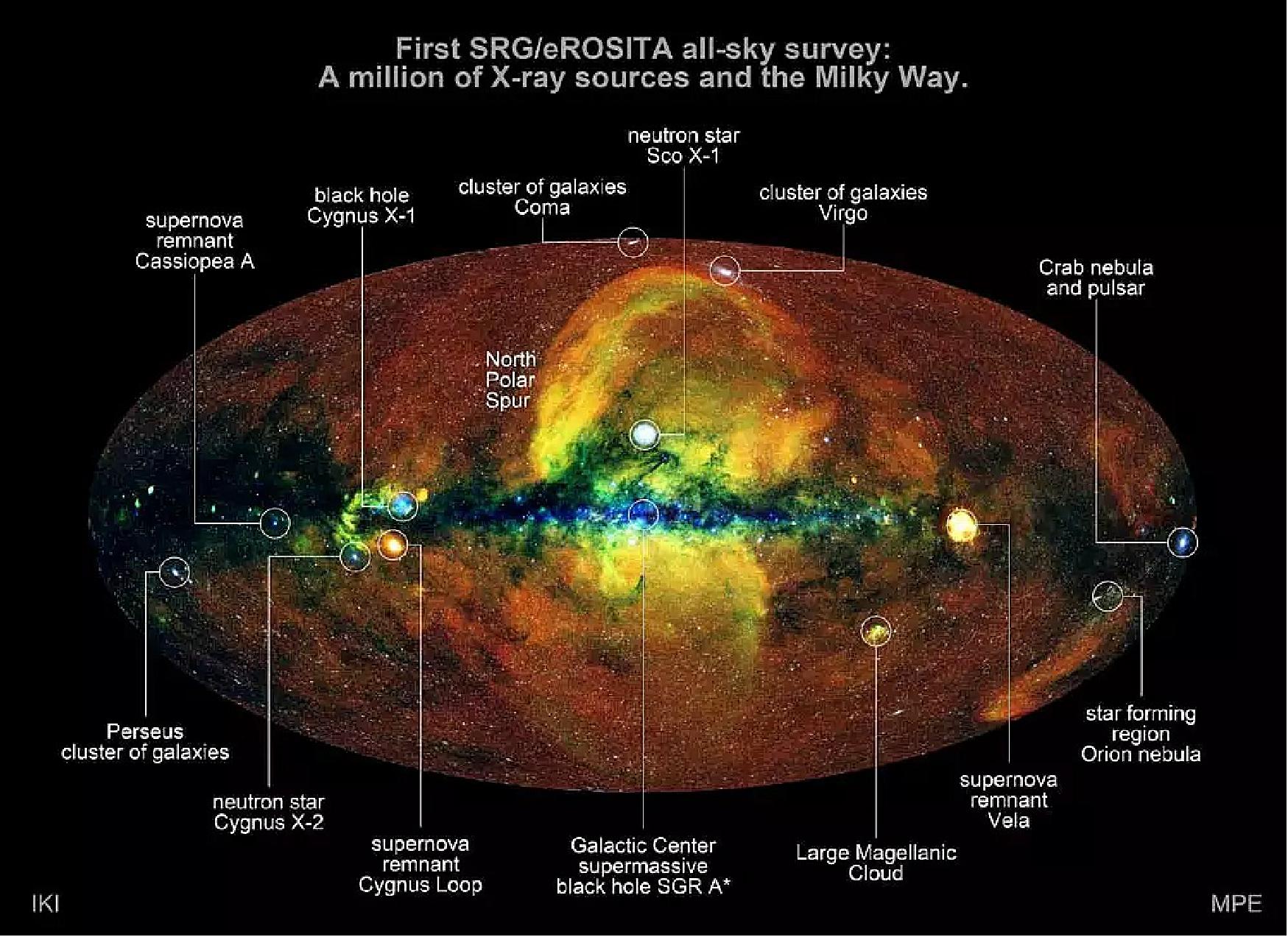
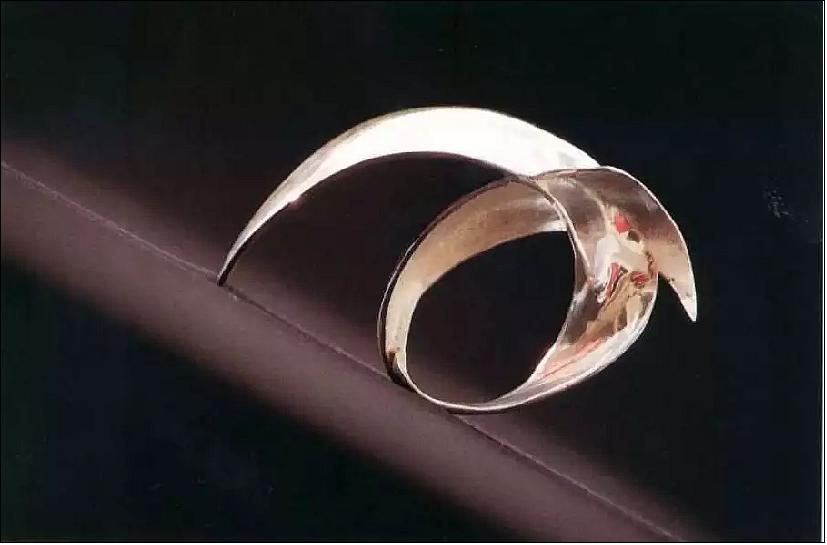
• May 17, 2021: By now, the SRG/eROSITA telescope has completed more than half of its third scan of the entire X-ray sky. The first all-sky image was released in summer 2020, which can be seen here in an interactive 360º view. This map contains more than one million objects, most of them active galactic nuclei at cosmological distances, marking the growth of gigantic black holes over cosmic time. Clusters of galaxies in the new map will be used to track the growth of cosmic structures and constrain cosmological parameters. Closer to home, stars with hot coronae, binaries and supernova remnants dot our Galaxy - the hot band in the middle of the image. 29)
- The good angular resolution (~ 30 arcseconds) and the high sensitivity of eROSITA made it possible to already map more than a million compact sources and tens of thousands of extended ones. Only the brightest of them are visible on the map as dots. The resolution of the map does not allow you to distinguish the rest of the objects individually; they merge, adding to the brightness of the diffuse radiation of the Galaxy and to the radiation of the extragalactic X-ray background.
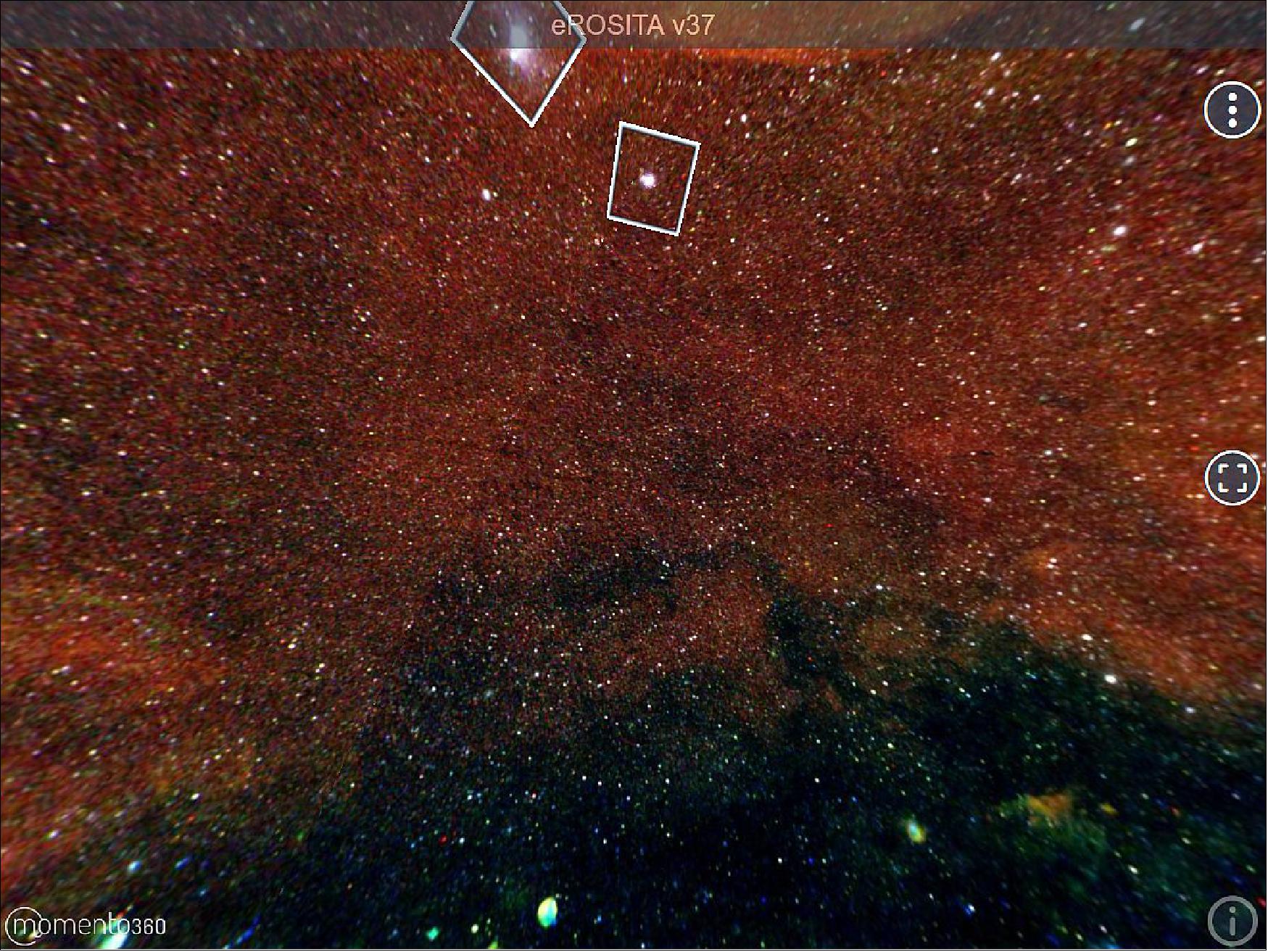
- The colors in this map where chosen to represent different energies of the incoming X-ray photons: Red corresponds to photons with energies of 0.3–0.6 keV, green to 0.6–1 keV, and blue to 1–2.3 keV. This – more or less – corresponds to a characteristic temperature of the radiating source: 3-6 million degrees (red), 6-10 million degrees (green), and 10-25 million degrees (blue).
• March 3, 2021: In the first all-sky survey by the eROSITA X-ray telescope onboard SRG, astronomers at the Max Planck Institute for Extraterrestrial Physics (MPE) have identified a previously unknown supernova remnant, dubbed “Hoinga”. The finding was confirmed in archival radio data and marks the first discovery of a joint Australian-eROSITA partnership established to explore our Galaxy using multiple wavelengths, from low-frequency radio waves to energetic X-rays. The Hoinga supernova remnant is very large and located far from the galactic plane – a surprising first finding – implying that the next years might bring many more discoveries. 30) 31)
- Massive stars end their lives in gigantic supernova explosions when the fusion processes in their interiors no longer produce enough energy to counter their gravitational collapse. But even with hundreds of billions of stars in a galaxy, these events are pretty rare. In our Milky Way, astronomers estimate that a supernova should happen on average every 30 to 50 years. While the supernova itself is only observable on a timescale of months, their remnants can be detected for about 100,000 years. These remnants are composed of the material ejected by the exploding star at high velocities and forming shocks when hitting the surrounding interstellar medium.
- About 300 such supernova remnants are known today – much less than the estimated 1200 that should be observable throughout our home Galaxy. So, either astrophysicists have misunderstood the supernova rate or a large majority has been overlooked so far. An international team of astronomers are now using the all-sky scans of the eROSITA X-ray telescope to look for previously unknown supernova remnants. With temperatures of millions of the degrees, the debris of such supernovae emits high-energy radiation, i.e. they should show up in the high-quality X-ray survey data.
- “We were very surprised that the first supernova remnant popped up straight away,” says Werner Becker at the Max Planck Institute for Extraterrestrial Physics. “Hoinga” is the largest supernova remnant ever discovered in X-rays. With a diameter of about 4.4 degrees, it covers an area about 90 times bigger than the size of the full Moon. “Moreover, it lies very far off the galactic plane, which is very unusual,” he adds. Most previous searches for supernova remnants have concentrated on the disk of our galaxy, where star formation activity is highest and stellar remnants therefore should be more numerous, but it seems that many supernova remnants have been overlooked by this search strategy.
- After the astronomers found the object in the eROSITA all-sky data, they turned to other resources to confirm its nature. Hoinga is – although barely – visible also in data taken by the ROSAT X-ray telescope 30 years ago, but nobody noticed it before due to its faintness and its location at high galactic latitude. However, the real confirmation came from radio data, the spectral band where 90% of all known supernova remnants were found so far. 32)
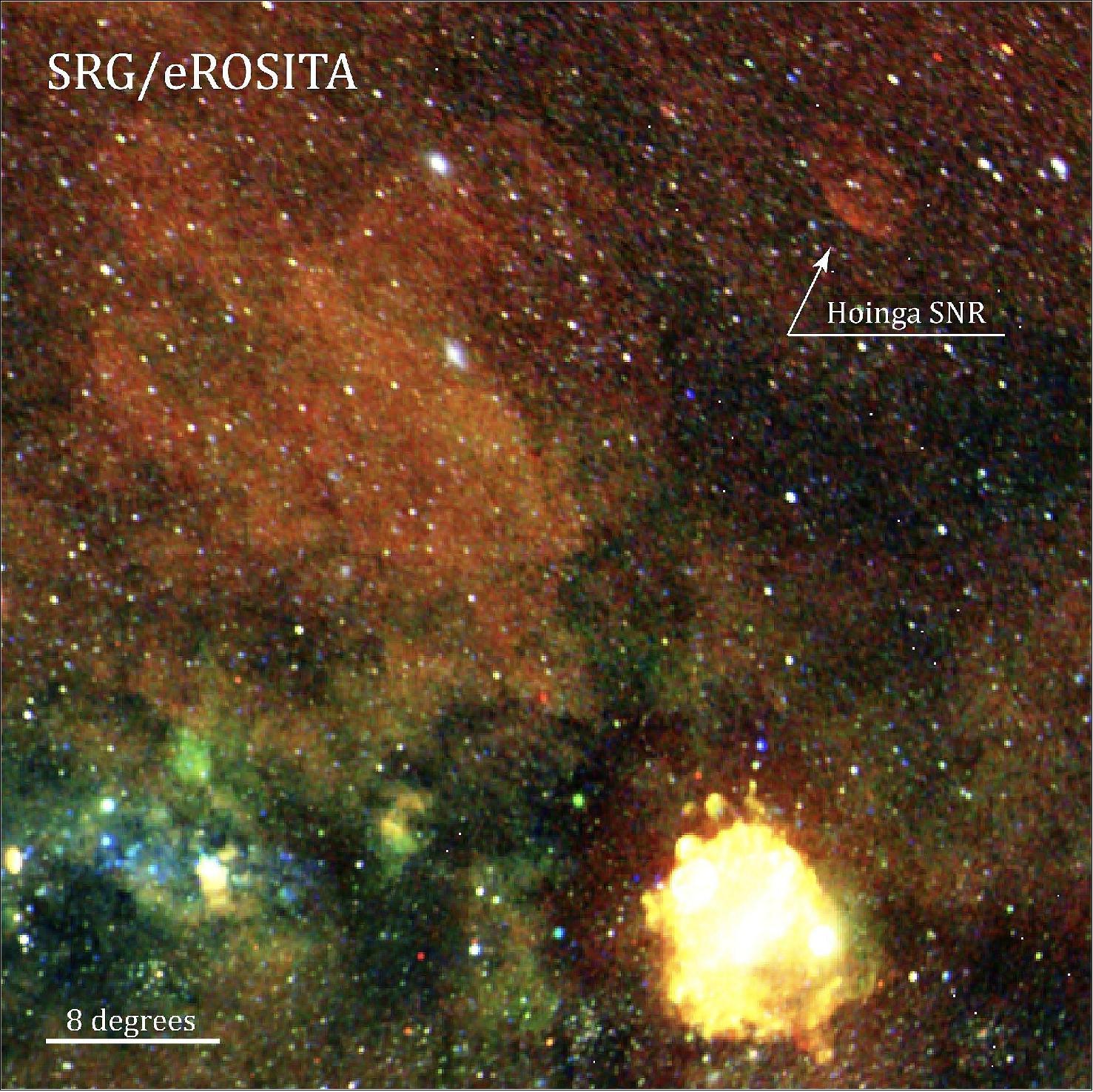
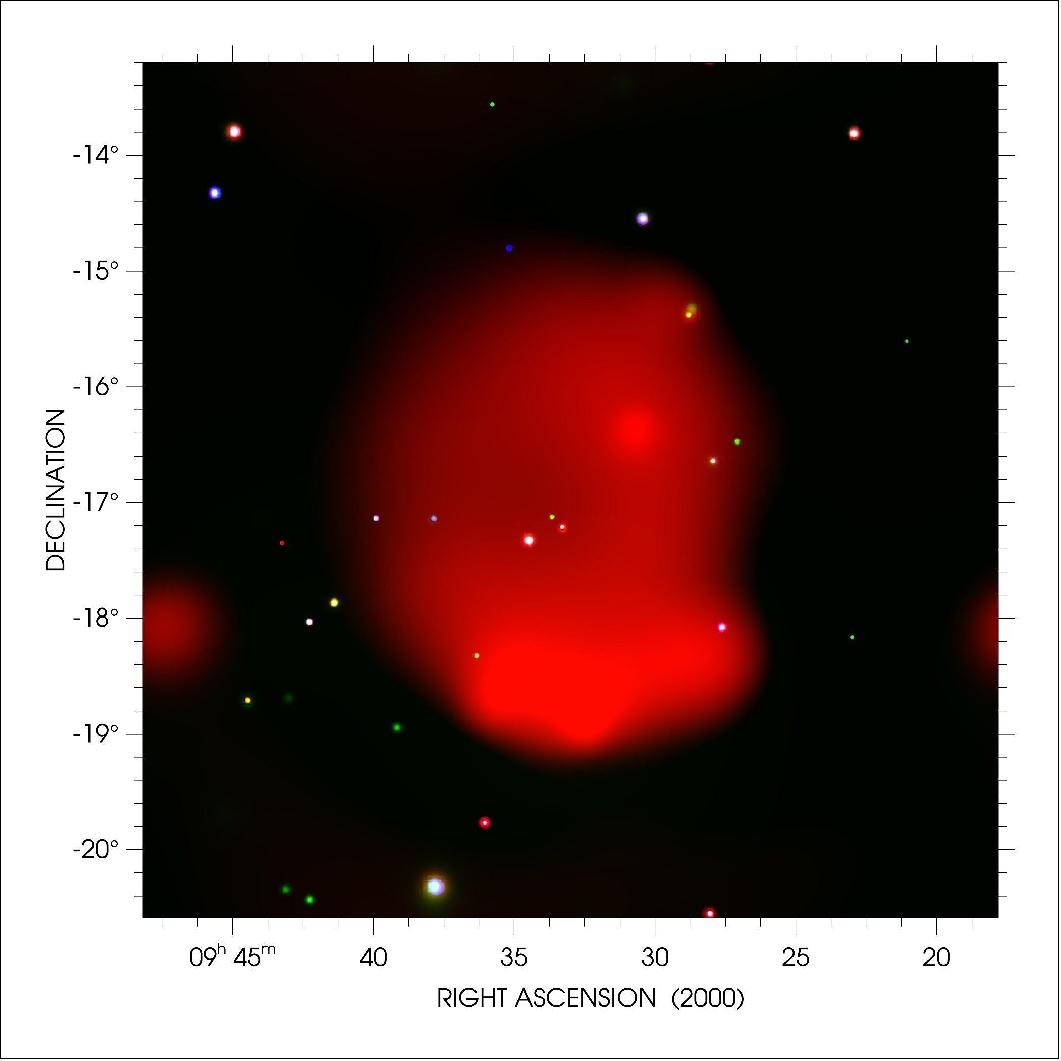
- “We went through archival radio data and it had been sitting there, just waiting to be discovered,” marvels Natasha Walker-Hurley, from the Curtin University node of the International Centre for Radio Astronomy Research in Australia. “The radio emission in 10-year-old surveys clearly confirmed that Hoinga is a supernova remnant, so there may be even more of these out there waiting for keen eyes.”
- The eROSITA X-ray telescope will perform a total of eight all-sky surveys and is about 25 times more sensitive than its predecessor ROSAT. Both observatories were designed, build and are operated by the Max Planck Institute for Extraterrestrial Physics. The astronomers expected to discover new supernova remnants in its X-ray data over the next few years, but they were surprised to identify one so early in the programme. Combined with the fact that the signal is already present in decades-old data, this implies that many supernova remnants might have been overlooked in the past due to low-surface brightness, being in unusual locations or because of other nearby emission from brighter sources. Together with upcoming radio surveys, the eROSITA X-ray survey shows great promise for finding many of the missing supernova remnants, helping to solve this long-standing astrophysical mystery.
![Figure 16: Composite X-ray and radio image of Hoinga. The X-rays discovered by eROSITA are emitted by the hot debris of the exploded progenitor, whereas the radio antennae detect synchrotron emission from relativistic electrons, which are decelerated at the outer remnant layer [image credit: eROSITA/MPE (X-ray), CHIPASS/SPASS/N. Hurley-Walker, ICRAR-Curtin (Radio)]](https://eoportal.org/ftp/satellite-missions/s/SpektrRG-SRG-020522/SpektrRG-SRG_Auto22.jpeg)
![Figure 17: Composite image of Hoinga taken at 1.4 GHz and 2.3 GHz by the CHIPASS and SPASS radio surveys. The blue color is arbitrary; fore- and background sources were removed from the images to increase the visibility of the diffuse radio emission from the supernova remnant [image credit: CHIPASS/SPASS/N. Hurley-Walker, ICRAR-Curtin (Radio)]](https://eoportal.org/ftp/satellite-missions/s/SpektrRG-SRG-020522/SpektrRG-SRG_Auto21.jpeg)
• December 9, 2020: The first all-sky survey performed by the eROSITA X-ray telescope on-board the Spektrum-Roentgen-Gamma (SRG) observatory has revealed a large hourglass-shaped structure in the Milky Way. These “eROSITA bubbles” show a striking similarity to the Fermi bubbles, detected a decade ago at even higher energies. The most likely explanation for these features is a massive energy injection from the Galactic center in the past, leading to shocks in the hot gas envelope of our galaxy. 33) 34)
- Astronomers have detected a remarkable new feature in the first all-sky survey map produced by the eROSITA X-ray telescope on SRG: a huge circular structure of hot gas below the plane of the Milky Way occupying most of the southern sky. A similar structure in the Northern sky, the “North polar spur”, has been known for a long time and had been thought to be the trace of an old supernova explosion. Taken together, the northern and the southern structures instead are reminiscent of a single hourglass-shaped set of bubbles emerging from the Galactic center.
- “Thanks to its sensitivity, spectral and angular resolution, eROSITA has been able to map the entire X-ray sky to unprecedented depth, revealing the southern bubble unambiguously,” explains Michael Freyberg, a senior scientist working on eROSITA at the Max Planck Institute for Extraterrestrial Physics (MPE). eROSITA scans the entire sky every six months and the data allows the scientists to look for structures that cover a significant portion of the whole sky.
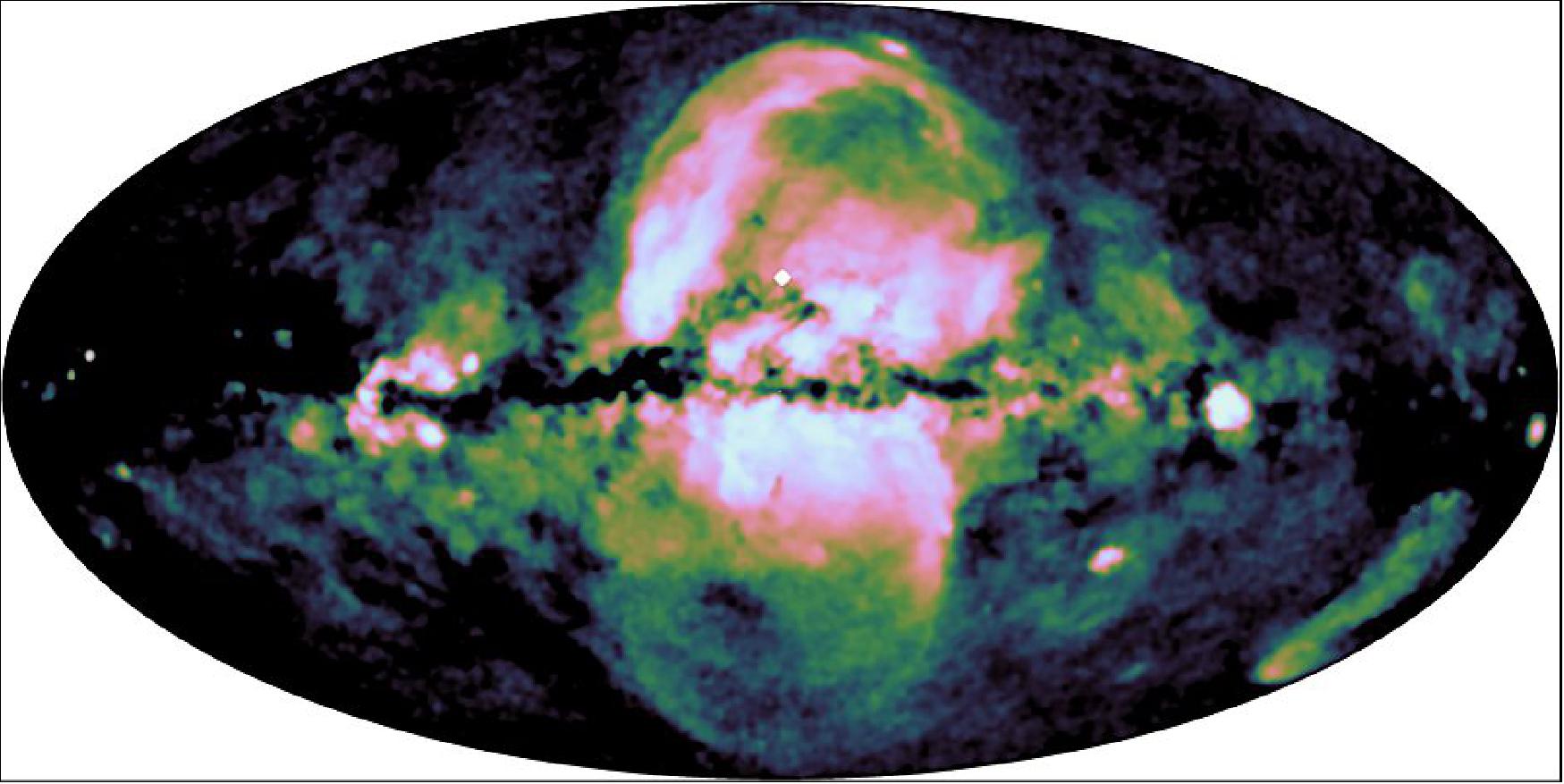
- The large-scale X-ray emission observed by eROSITA in its medium energy band (0.6-1.0 keV) show that the intrinsic size of the bubbles is several kiloparsecs (or up 50,000 light-years) across, almost as large as the entire Milky Way. These ‘eROSITA bubbles’ show striking morphological similarities to the well-known ‘Fermi bubbles’ detected at in gamma-rays by the Fermi telescope, but are larger and more energetic.
- „The sharp boundaries of these bubbles most likely trace shocks caused by the massive injection of energy from the inner part of our galaxy into the Galactic halo,” points out Peter Predehl, first author of the study now published in Nature. “Such an explanation has been previously suggested for the Fermi bubbles, and now with eROSITA their full extent and morphology has become evident.”
- This discovery will help astronomers to understand the cosmic cycle of matter in and around the Milky Way, and other galaxies. Most of the ordinary (baryonic) matter in the Universe is invisible to our eyes, with all the stars and galaxies that we observe with optical telescopes comprising less than 10% of its total mass. Vast amounts of unobserved baryonic matter are expected to reside in tenuous haloes wrapped like cocoons around the galaxies and the filaments between them in the cosmic web. These haloes are hot, with a temperature of millions of degrees, and thus only visible with telescopes sensitive to high-energy radiation.
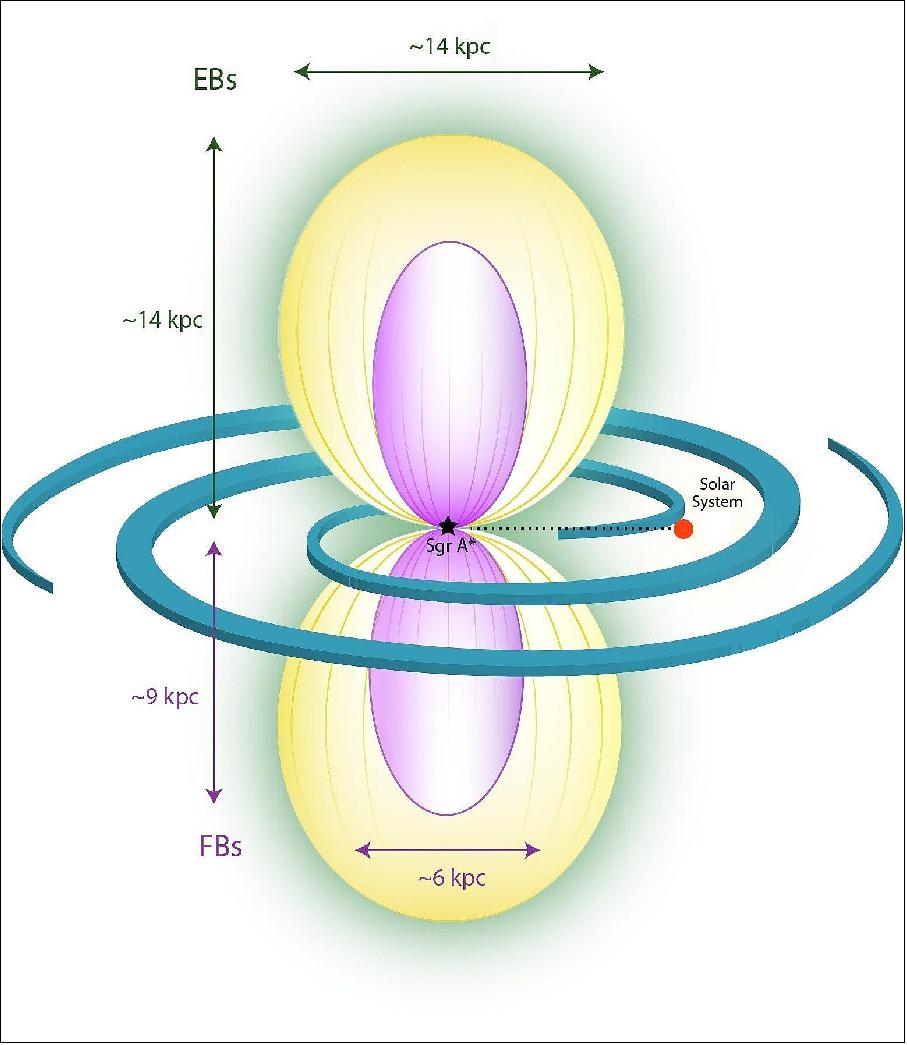
- The bubbles now seen with eROSITA trace disturbances in this hot gas envelope around our Milky Way, caused either by a burst of star formation or by an outburst from the supermassive black hole at the Galactic centre. While dormant now, the black hole could well have been active in the past, linking it to active galactic nuclei (AGN) with rapidly growing black holes seen in distant galaxies. In either case, the energy needed to power the formation of these huge bubbles must have been enormous at 1056 ergs, equivalent to the energy release of 100,000 supernovae, and similar to estimates of AGN outbursts.
- “The scars left by such outbursts take a very long time to heal in these haloes,” adds Andrea Merloni, eROSITA Principal Investigator. “Scientists have been looking for the gigantic fingerprints of such past violent activity around many galaxies in the past.” The eROSITA bubbles now provide strong support for large-scale interactions between the Galaxy core and the halo around it, which are energetic enough to perturb the structure, energy content and chemical enrichment of the circumgalactic medium of the Milky Way.
- “eROSITA is currently completing the second scan of the entire sky, doubling the number of X-ray photons coming from the bubbles it has discovered,” points out Rashid Sunyaev, Lead Scientist of the SRG Observatory in Russia. “We have a tremendous amount of work ahead of us, because the eROSITA data makes it possible to single out many X-ray spectral lines emitted by highly ionized gas. This means that the door is open to study the abundance of chemical elements, the degree of their ionization, the density and temperature of the emitting gas in the bubbles, and to identify the locations of shock waves and estimate characteristic timescales.”
• June 24, 2020: European and Russian specialists recently worked together to catch signals from an astrophysical observatory mission, now mapping X-ray sources in our galaxy and beyond, discovering previously unknown supermassive black holes. 35)
- In a joint technology demonstration conducted in April and May, ESA, Roscosmos, and the Space Research Institute of the Russian Academy of Sciences (IKI RAN) in cooperation with NPO Lavochkin used three of the European Space Agency’s deep space ground stations to download vital scientific data from the Spektr-RG spacecraft.
- The Spektr-RG astrophysical observatory was developed by Roscosmos with participation from Germany led by the German Aerospace Center (DLR), and is currently operating in a halo orbit around a special point in space called the L2 Lagrange point, some 1.5 million km from Earth. From here, its goal is to map the entire sky in X-rays and identify new X-ray sources, such as supermassive black holes, across the Universe.
ESA stations link up
- This spring, the Russian ground stations normally used to communicate with Spektr-RG were in unfavorable geographic positions, and experts from ESA’s Estrack ground station network stepped in, working in close cooperation with colleagues at the Russian Complex for Receiving Scientific Information (RKPNI).
- ESA’s three 35 m dish antennas, located in Australia, Spain and Argentina, were used to establish a series of 16 communication passes with Spektr-RG, downloading 6.5 GB of science data.
- These included imagery generated by the mission’s two X-ray telescopes: the ART-XC instrument developed by IKI RAN and the eROSITA instrument, built and operated by the Max Planck Institute for Extraterrestrial Physics (MPE) in Germany.
- It was the first time that the Agency’s ground stations collected scientific data from a Russian spacecraft.
![Figure 20: These eROSITA images show the two interacting galaxy clusters A3391, towards the top of the images, and the bimodal cluster A3395, towards the bottom, highlighting eROSITA’s excellent view of the distant Universe. They were observed in a series of image acquisitions performed between 17 and 18 October 2019, using all seven eROSITA telescope modules [image credit: T. Reiprich (Univ. Bonn), M. Ramos-Ceja (MPE), F. Pacaud (Univ. Bonn), D. Eckert (Univ. Geneva), J. Sanders (MPE), N. Ota (Univ. Bonn), E. Bulbul (MPE), V. Ghirardini (MPE), MPE/IKI] 36)](https://eoportal.org/ftp/satellite-missions/s/SpektrRG-SRG-020522/SpektrRG-SRG_Auto1E.jpeg)
• June 19, 2020: Over the course of 182 days, the eROSITA X-ray telescope onboard SRG has completed its first full sweep of the sky. This new map of the hot, energetic universe contains more than one million objects, roughly doubling the number of known X-ray sources discovered over the 60-year history of X-ray astronomy. Most of the new sources are active galactic nuclei at cosmological distances, marking the growth of gigantic black holes over cosmic time. Clusters of galaxies in the new map will be used to track the growth of cosmic structures and constrain cosmological parameters. Closer to home, stars with hot coronae, binaries and supernova remnants dot our Galaxy, and we now have a complete map of the hot baryons in the Milky Way, something that can only be achieved with the 360-degree view provided by the eROSITA survey. 37) 38)
![Figure 21: The energetic universe as seen with the eROSITA X-ray telescope. The first eROSITA all-sky survey was conducted over a period of six months by letting the telescope rotate continuously, thus providing a uniform exposure of about 150-200 seconds over most of the sky, with the ecliptic poles being visited more deeply. As eROSITA scans the sky, the energy of the collected photons is measured with an accuracy ranging from 2% - 6%. To generate this image, in which the whole sky is projected onto an ellipse (so-called Aitoff projection) with the center of the Milky Way in the middle and the body of the Galaxy running horizontally, photons have been color-coded according to their energy (red for energies 0.3-0.6 keV, green for 0.6-1 keV, blue for 1-2.3 keV). The original image, with a resolution of about 10”, and a corresponding dynamic range of more than one billion, is then smoothed (with a 10' FWHM Gaussian) in order to generate the above picture. The red diffuse glow away from the galactic plane is the emission of the hot gas in the vicinity of the solar system (the Local Bubble). Along the plane itself, dust and gas absorb the lowest energy X-ray photons, so that only high-energy emitting sources can be seen, and their color appears blue in the image. The hotter gas close to the galactic center, shown in green and yellow, carries imprinted the history of the most energetic processes in the life of the Milky Way, such as supernova explosions, driving fountains of gas out of the plane, and, possibly, past outburst from the now dormant supermassive black hole in the center of the galaxy. Piercing through this turbulent, hot diffuse medium, are hundreds of thousands of X-ray sources, which appear mostly white in the image, and uniformly distributed over the sky. Among them, distant active galactic nuclei (including a few emitting at a time when the Universe was less than one tenth of its current age) are visible as point sources, while clusters of galaxies reveal themselves as extended X-ray nebulosities. In total, about one million X-ray sources have been detected in the eROSITA all-sky image, a treasure trove that will keep the teams busy for the coming years [image credit: Jeremy Sanders, Hermann Brunner and the eSASS team (MPE); Eugene Churazov, Marat Gilfanov (on behalf of IKI)]](https://eoportal.org/ftp/satellite-missions/s/SpektrRG-SRG-020522/SpektrRG-SRG_Auto1D.jpeg)
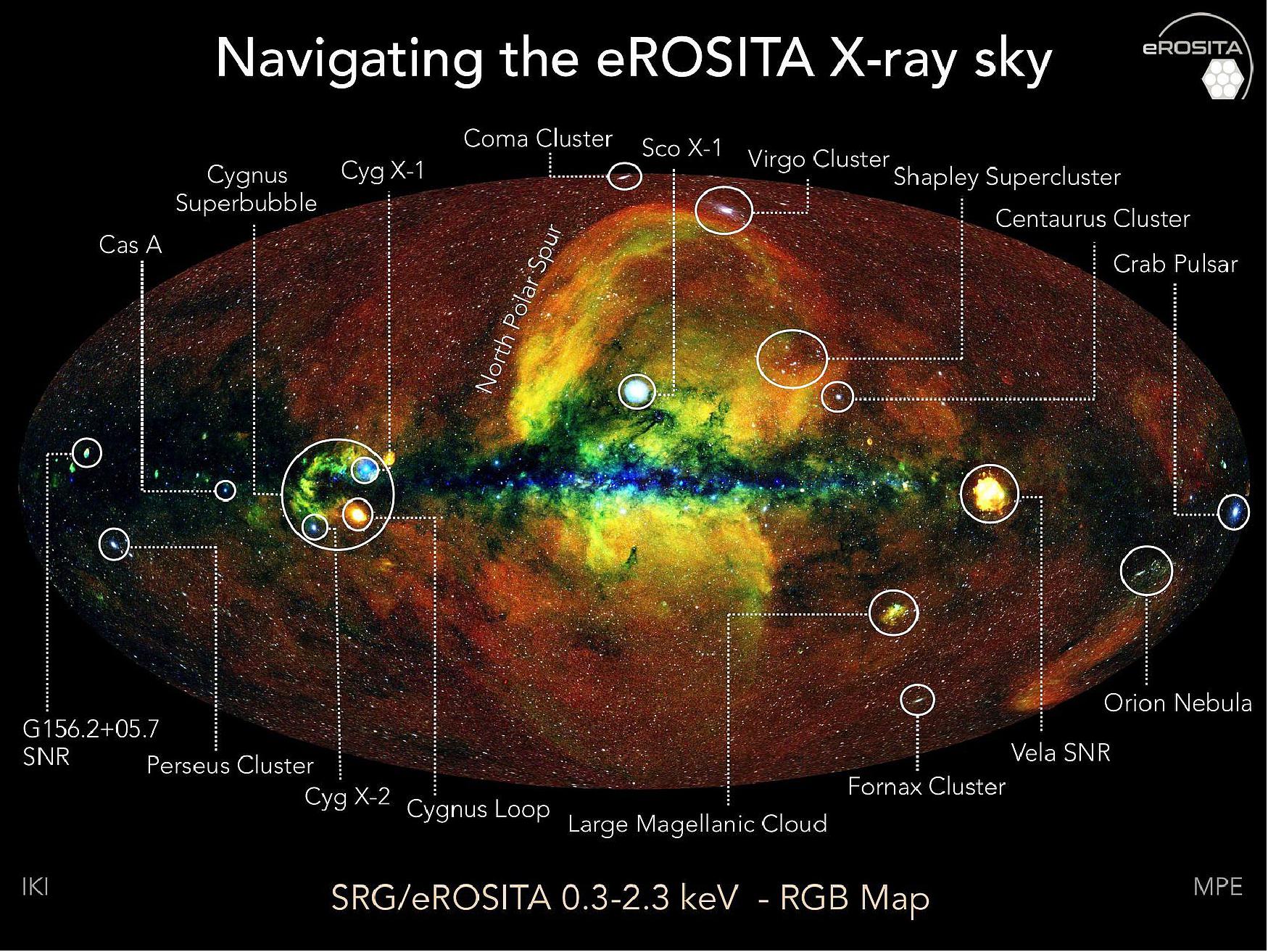
- A million X-ray sources revealing the nature of the hot universe – this is the impressive harvest of the first scan of the entire sky with the eROSITA telescope onboard SRG. “This all-sky image completely changes the way we look at the energetic universe,” says Peter Predehl, the Principal Investigator of eROSITA at the Max Planck Institute for Extraterrestrial Physics (MPE). “We see such a wealth of detail - the beauty of the images is really stunning.”
- This first complete sky image from eROSITA is about 4 times deeper than the previous all-sky survey by the ROSAT telescope 30 years ago, and has yielded around 10 times more sources: about as many as have been discovered by all past X-ray telescopes combined. And while most classes of astronomical objects emit in X-rays, the hot and energetic Universe looks quite different to the one seen by optical or radio telescopes. Looking outside the body of our Galaxy, most of the eROSITA sources are active galactic nuclei, accreting supermassive black holes at cosmological distances, interspersed with clusters of galaxies, which appear as extended X-ray haloes shining thanks to the hot gas confined by their huge concentrations of dark matter. The all-sky image reveals in exquisite detail the structure of the hot gas in the Milky Way itself, and the circum-galactic medium, which surrounds it, whose properties are key to understanding the formation history of our Galaxy. The eROSITA X-ray map also reveals stars with strong, magnetically active hot coronae, X-ray binary stars containing neutron stars, black holes or white dwarves, and spectacular supernova remnants in our own and other nearby galaxies such as the Magellanic clouds.
- "We were all eagerly awaiting the first all-sky map from eROSITA,” says Mara Salvato, the scientist at MPE who leads the effort to combine eROSITA observations with other telescopes across the electromagnetic spectrum. “Large sky areas have already been covered at many other wavelengths, and now we have the X-ray data to match. We need these other surveys to identify the X-ray sources and understand their nature.” The survey is also a treasure trove of rare and exotic phenomena, including numerous types of transients and variables, such as flares from compact objects, merging neutron stars, and stars being swallowed by black holes. “eROSITA often sees unexpected bursts of X-rays from the sky,” continues Salvato. “We need to alert ground-based telescopes immediately to understand what’s producing them.”
- Assembling the image has been a mammoth task. So far, the operations team has received and processed about 165 GB of data collected by eROSITA’s seven cameras. While relatively small by “big-data” standards on the ground, operating this complex instrument in space provided its own special challenges. “We check and monitor the health of the instrument on a daily basis, in cooperation with our colleagues in Moscow who operate the SRG spacecraft” explains Miriam Ramos-Ceja, a member of the eROSITA operations team at MPE. “This means we can respond quickly to any anomalies. We’ve been able to react to these immediately to keep the instrument safe, while collecting data at ~97% efficiency. It’s amazing to be able to communicate in real time with an instrument located 1.5 million kilometers away!” The data downlink occurs daily. “We perform immediate quality checks on the data”, she continues, “before it is being processed and analyzed by the teams in Germany and Russia.”
- While the team is now busy analyzing this first all-sky map and using the images and catalogues to deepen our understanding of cosmology and high-energy astrophysical processes, the telescope continues its sweep of the X-ray sky. “The SRG Observatory is now starting its second all-sky survey, which will be completed by the end of this year“, says Rashid Sunyaev, Lead Scientist of the Russian SRG team. “Overall, during the next 3.5 years, we plan to get 7 maps similar to the one seen in this beautiful image. Their combined sensitivity will be a factor of 5 better and will be used by astrophysicists and cosmologists for decades.“
- Kirpal Nandra, head of the high-energy astrophysics group at MPE, adds “With a million sources in just six months, eROSITA has already revolutionized X-ray astronomy, but this is just a taste of what’s to come. This combination of sky area and depth is transformational. We are already sampling a cosmological volume of the hot Universe much larger than has been possible before. Over the next few years, we’ll be able to probe even further, out to where the first giant cosmic structures and supermassive black holes were forming.”
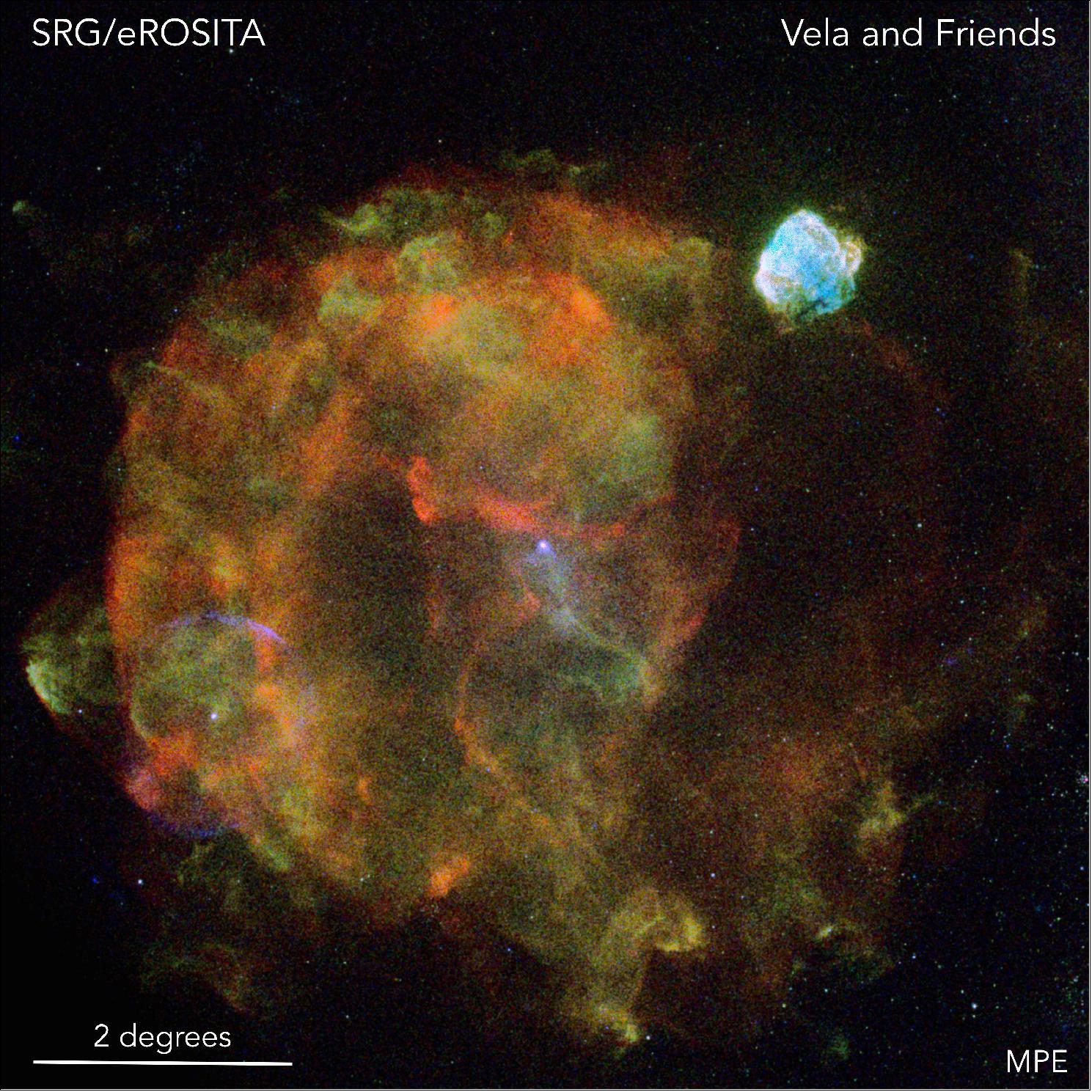
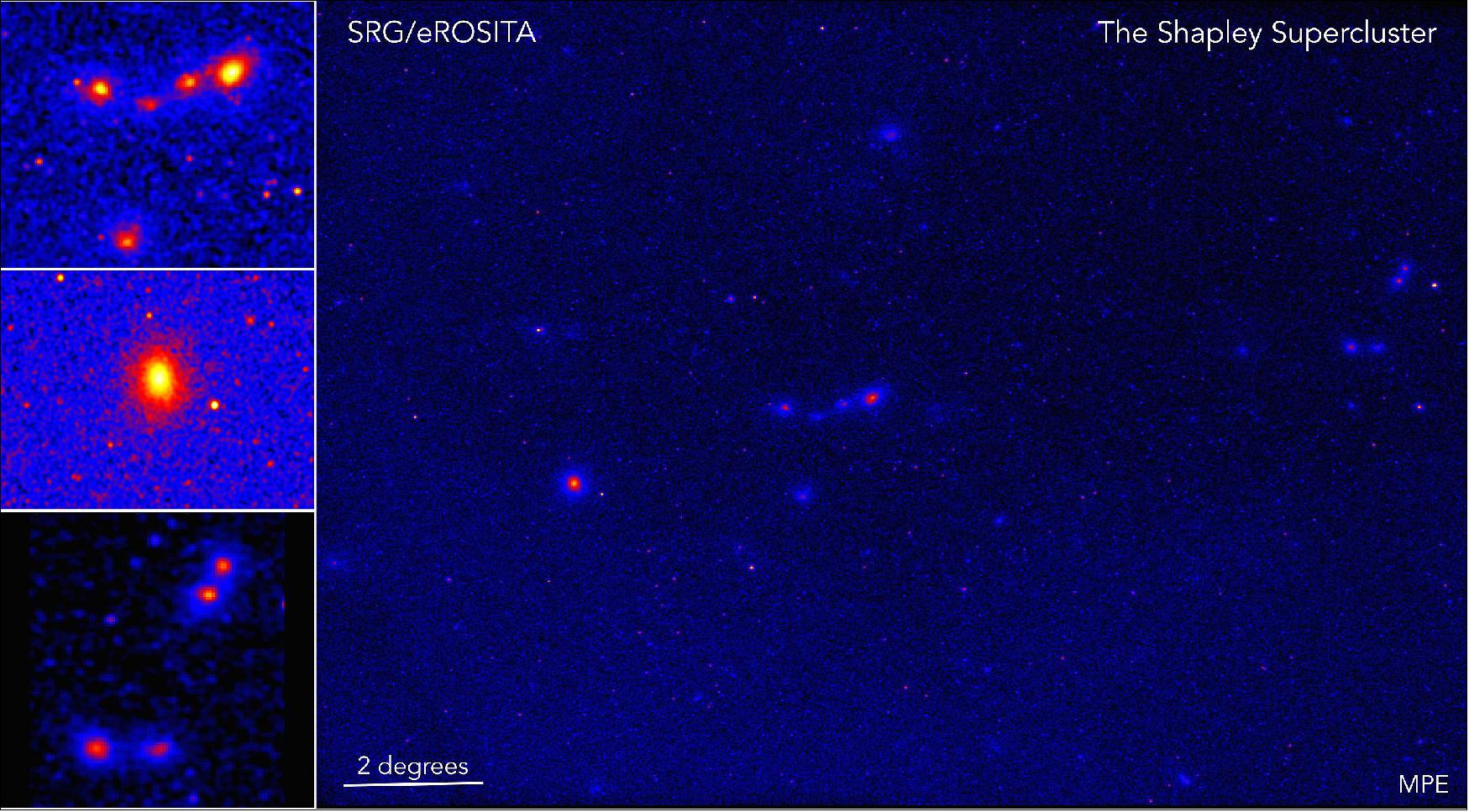
• June 11, 2020: The Russian-German Space Observatory Spektr-RG has completed the first-ever all-sky hard X-ray survey, the Space Research Institute (IKI) of the Russian Academy of Sciences said. 39)
- "We have made it – the ART-XC telescope of the Spektr-RG observatory has completed its first all-sky survey! As we have predicted, it took almost a half of the year, during which the telescope was permanently monitoring the sky in hard X-rays", the institute said on Wednesday.
- The telescope has been scanning the celestial sphere in hard X-rays from 8 December 2019 till 10 June 2020, the institute's news release reads. The observatory has registered all space events in the energy range 4–12 kiloelectron volt (keV).
- The institute said that the all-sky map with a similar resolution had been created by the German observatory ROSAT in soft X-rays 30 years ago. Other sky hard X-ray maps have a much worse resolution that the map prepared by Spektr-RG.
- The Spektr-RG mission is a joint project between Roscosmos and the German space agency DLR. Spektr-RG was launched from the Baikonur Cosmodrome in Kazakhstan on 13 July 2019.
- For the next four years, the telescope is expected to perform eight all-sky surveys to map X-rays sources in the universe with unprecedented detail.
- X-ray telescopes are used for photographing distant objects of the universe, such as clusters of galaxies, active galactic nuclei, supernova remnants, and x-ray binary stars. The difficulty of observation in the x-ray range is that the telescope must be raised above the atmosphere of the earth, opaque to x-rays.
• January 13, 2020: Using ESA’s XMM-Newton X-ray observatory, scientists led by the Max Planck Institute for Extraterrestrial Physics have identified hot gas sloshing around inside a galaxy cluster – a never-before-seen behavior that may be driven by turbulent merger events. 40) 41)
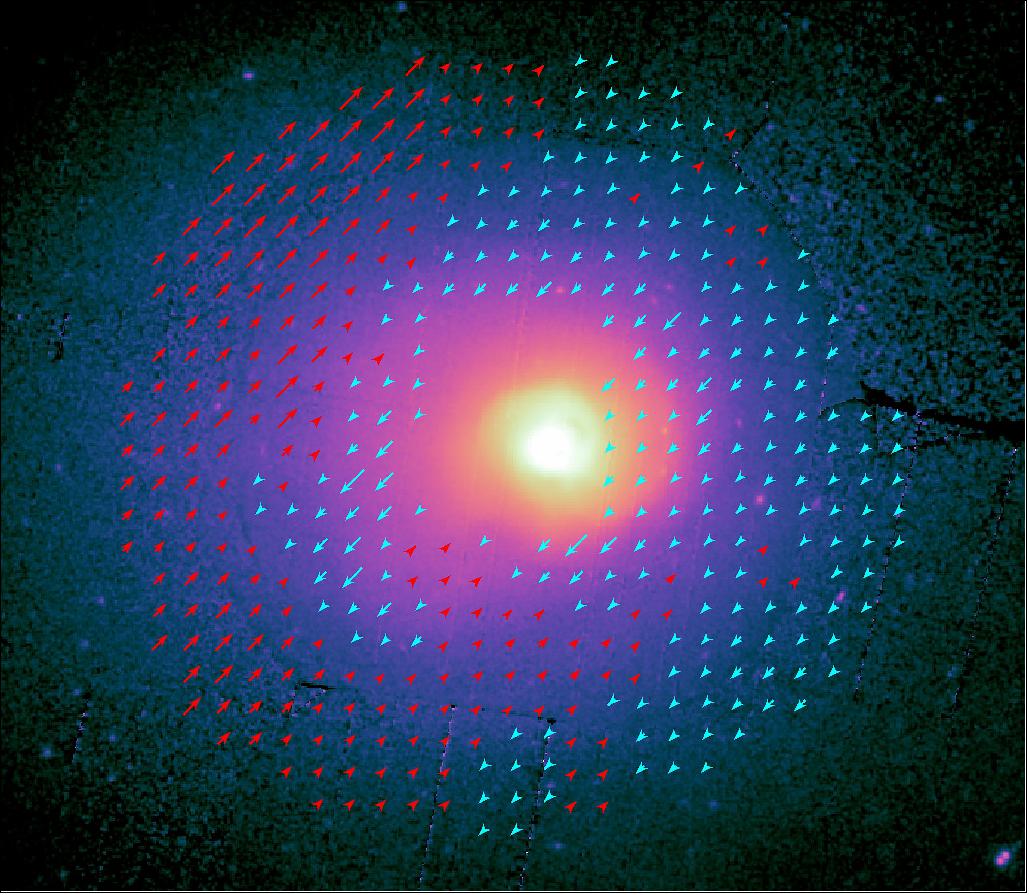
- Galaxy clusters are the largest systems in the Universe bound together by gravity. They contain hundreds to thousands of galaxies and large quantities of hot gas known as plasma, which reaches temperatures of around 50 million degrees and shines brightly in X-rays. Very little is known about how this plasma moves, but exploring its motions may be key to understanding how galaxy clusters form, evolve and behave.
- “We selected two nearby, massive, bright and well-observed galaxy clusters, Perseus and Coma, and mapped how their plasma moved – whether it was moving towards or away from us, its speed, and so on – for the first time,” says lead author Jeremy Sanders of the Max Planck Institute for Extraterrestrial Physics (MPE) in Garching, Germany. “We did this over large regions of sky: an area roughly the size of two full Moons for Perseus, and four for Coma.”
- The astronomers found direct signs of plasma flowing, splashing and sloshing around within the Perseus galaxy cluster – one of the most massive known objects in the Universe, and the brightest cluster in the sky in terms of X-rays. While this kind of motion has been predicted theoretically, it had never been seen before in the cosmos.
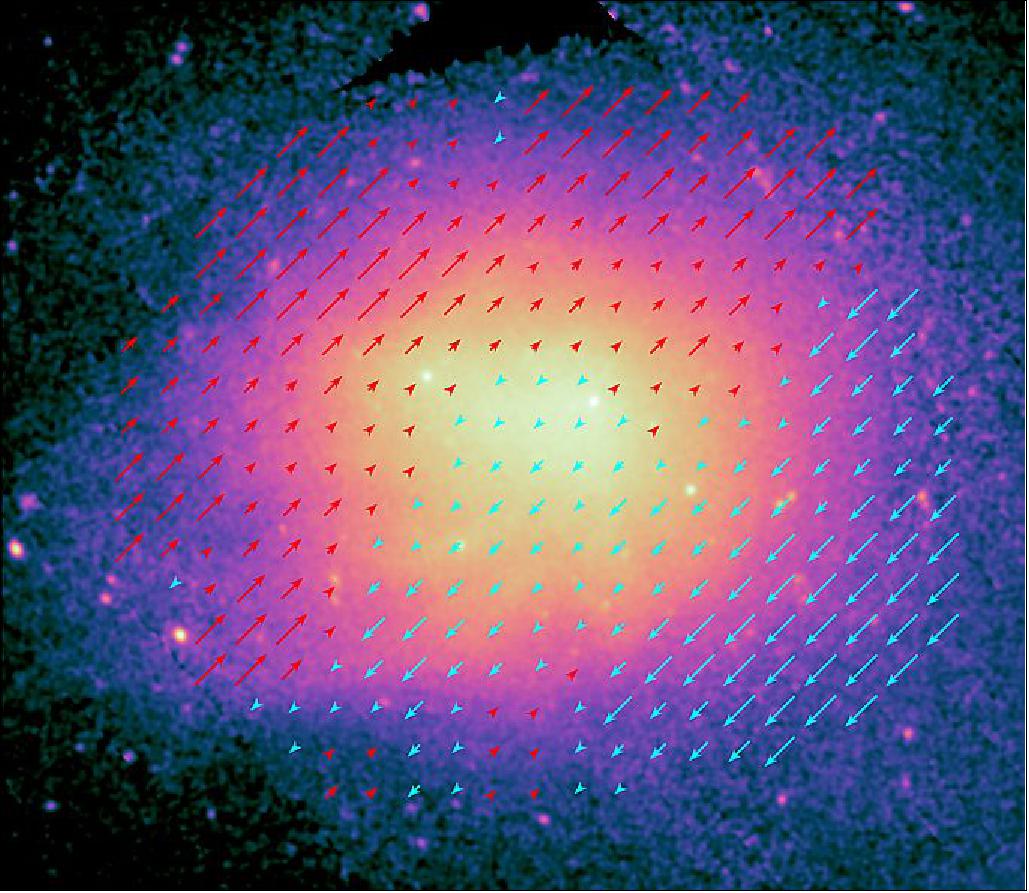
- By looking at simulations of how the plasma moved within the cluster, the researchers then explored what was causing the sloshing. They found it to be likely due to smaller sub-clusters of galaxies colliding and merging with the main cluster itself. These events are energetic enough to disrupt Perseus’ gravitational field and kickstart a sloshing motion that will last for many millions of years before settling.
- Unlike Perseus, which is characterized by a main cluster and several smaller sub-structures, the Coma cluster contained no sloshing plasma, and appears to instead be a massive cluster made up of two major sub-clusters that are slowly merging together.
- “Coma contains two massive central galaxies rather than a cluster’s usual single behemoth, and different regions appear to contain material that moves differently,” says Sanders. “This indicates that there are multiple streams of material within the Coma cluster that haven’t yet come together to form a single coherent ‘blob’, like we see with Perseus.”
• December 8, 2019: Launched from Baikonur on 13 July 2019 to the second Sun-Earth Lagrange point (L2), the Russian-German SRG mission has now started its main task. On December 8th, after an extensive program of commissioning, calibration and performance verification of its two X-ray telescopes (ART-XC and eROSITA), the satellite has begun observing the sky in continuous scanning mode. As SRG follows the revolution of Earth, and hence also of the L2 point, around the Sun, it will perform eight complete surveys of the whole sky, one every six months, for the next 4 years. Pre-launch predictions suggest that, over that time, the eROSITA instrument, conceived, designed and built at MPE, should discover approximately 100,000 clusters of galaxies, around 3 million accreting supermassive black holes and half a million active stars. 42)
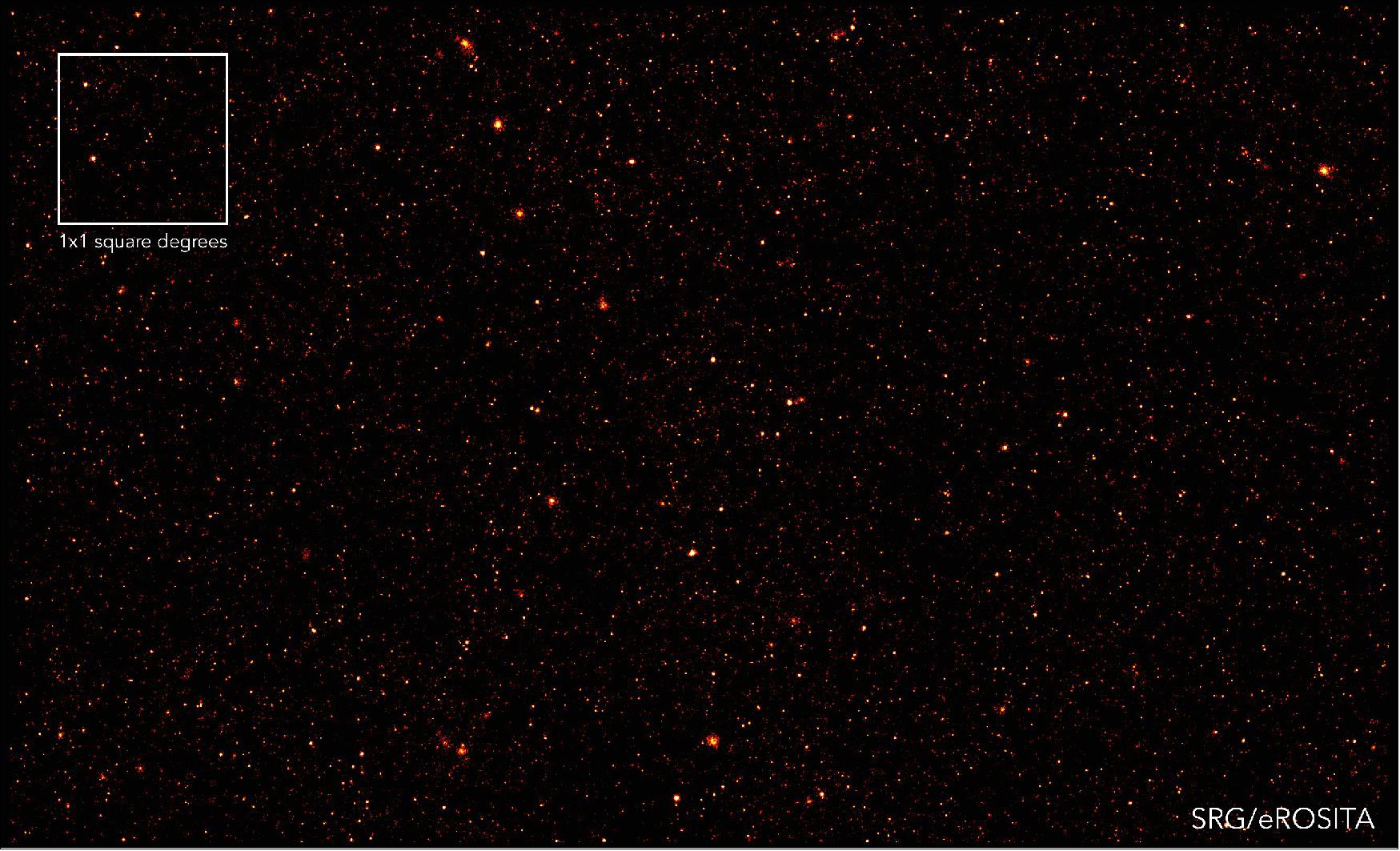
- To confirm those predictions for the all-sky survey, eROSITA scientists have performed a number of test observations over the past few weeks. Among those, a mini-survey called eFEDS (eROSITA Final Equatorial Depth Survey) was devised in order to image a small patch of the sky to the same depth expected at the end of the 4-years all-sky survey. The eFEDS data show the same stunning image quality demonstrated by the eROSITA first light (see also IKI press-release featuring more eROSITA images of the Lockman Hole and the Galactic Plane). More importantly, they allowed the scientists to confirm with great accuracy the sensitivity of the X-ray telescope to its main target classes.
- Over an area of just 1/300 of the full sky, eROSITA revealed more than 18,000 point-like X-ray sources, around 85% of them being distant Active Galactic Nuclei (AGN) harboring growing supermassive black holes, and most of the remainder X-ray stars. The mini-survey also discovered more then 400 clusters of galaxies (including a few at a redshift around 1), easily recognizable from their extended, diffuse morphology in the sharp X-ray images (Figure 28).
• On 22 October 2019, the beautiful first X-ray images of the eROSITA telescope were presented to the public at the Max Planck Institute for Extraterrestrial Physics (MPE) in Garching. After an extended commissioning phase, since October 13 all seven X-ray telescope modules with their custom-designed CCD cameras have been observing the sky simultaneously. The first combined X-ray images of our neighboring galaxy, the Large Magellanic Cloud, and a pair of interacting clusters of galaxies at a distance of about 800 million lightyears, show remarkable details and demonstrate the promise of the ambitious science program planned with the spaceborne telescope. 43)
- “These first images from our telescope show the true beauty of the hidden Universe,” enthuses Peter Predehl, Principal Investigator of eROSITA. “To meet our science goals we needed enough sensitivity to detect the most distant clusters of galaxies in the Universe over the whole sky, and resolve them spatially. These First Light images show that we can do exactly that, but we can go a lot further.” In addition to the sharp X-ray vision provided by each of eROSITA’s seven mirrors, each telescope is equipped with state-of-the-art CCD cameras with superb spectral and timing resolution. “The potential for new discoveries is immense. Now we can start reaping the fruits of more than ten years of work,” adds Predehl.
- The eROSITA ‘First Light’ images were obtained in a series of exposures of all seven telescope modules with a combined integration time of about one day for both the Large Magellanic Cloud (LMC), our neighboring galaxy, and the A3391/3395 system of interacting clusters of galaxies at a distance of about 800 million lightyears.
- In our neighboring galaxy, the LMC, eROSITA not only shows the distribution of the diffuse hot gas, but also some remarkable details, such as supernova remnants like SN1987A. The eROSITA image now confirms that this source is becoming fainter, as the shock wave produced by the stellar explosion in 1987 propagates through the interstellar medium. In addition to a host of other hot objects in the LMC itself, eROSITA also reveals a number of foreground stars from our own Milky Way galaxy as well as distant Active Galactic Nuclei, whose radiation pierces the diffuse emission of the hot gas in the LMC.
- “X-rays give us a unique view of the Universe, hidden in visible light”, explains Kirpal Nandra, director of High Energy astrophysics at MPE. “Looking at an apparently normal star, in X-rays we might see an orbiting white dwarf or neutron star in the process of devouring its companion. Visible light shows the structure of a galaxy traced by its stars, but the X-rays are dominated by supermassive black holes growing at their centers. And where we see clusters of galaxies with optical telescopes, X-rays reveal the huge reservoirs of gas filling the space between them and tracing out the dark matter structure of the Universe. With its performance demonstrated, we now know that eROSITA will lead to a breakthrough in our understanding of the evolution of the energetic Universe."
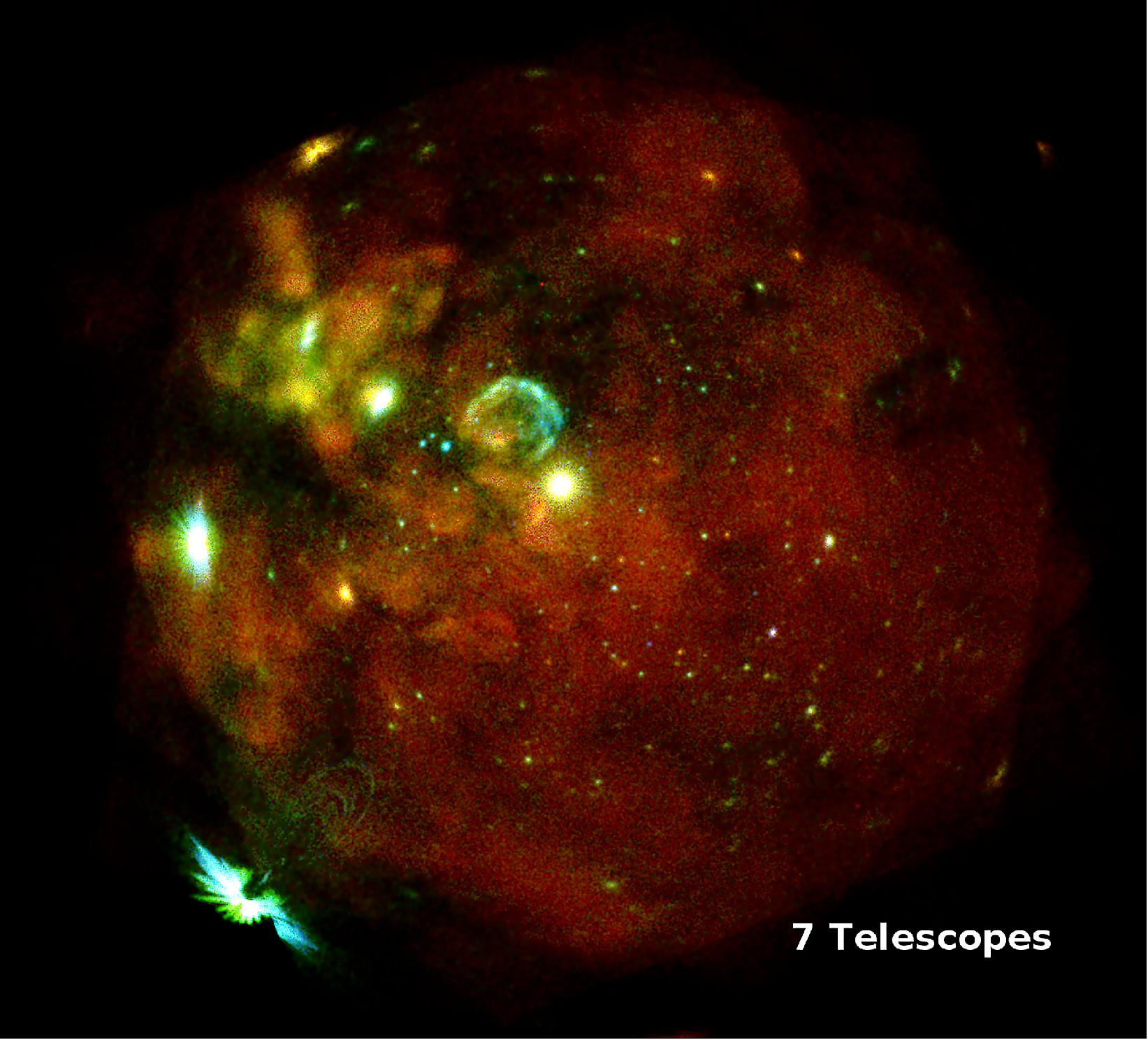
- Reaching further out into the Universe, the eROSITA image of the A3391/3395 system of interacting clusters of galaxies highlights the dynamical processes which lead to the formation of gigantic structures in the Universe. The clusters, appearing as large, elliptical nebulae in the eROSITA images, span tens of millions of lightyears across, and contain thousands of galaxies each. Clusters are one of the main science targets for eROSITA; astronomers expect to find some 100,000 X-ray emitting galaxy clusters as well as several million active black holes in the centers of galaxies during its 4-year all-sky survey in the soft and hard X-ray bands. “By measuring the evolution of these clusters over cosmic time, we can make precision measurements of the cosmological parameters to better understand the dark matter and dark energy that dominate the Universe” says astrophysicist Esra Bulbul, who leads the work on clusters and cosmology at MPE.
![Figure 29: These two eROSITA images show the two interacting galaxy clusters A3391, to the top of the image, and the double-peaked cluster A3395, to the bottom, highlighting eROSITA’s superb view of the distant Universe. They were observed in a series of exposures with all seven eROSITA telescope modules taken from 17 to 18 October 2019. The individual images were subjected to different analysis techniques, and then colored in different schemes to highlight the different structures. In the left-hand image, the red, green and blue colors refer to the three different energy bands of eROSITA. One clearly sees the two clusters as nebulous structures, which shine brightly in X-rays due to the presence of extremely hot gas (tens of millions of degrees) in the space between galaxies. The image on the right highlights the “bridge” or “filament” between the two clusters, confirming the suspicion that these two huge structures do interact dynamically. The eROSITA observations also show hundreds of point-like sources, signposting either distant supermassive black holes or hot stars in the Milky Way [image credit: T. Reiprich (Univ. Bonn), M. Ramos-Ceja (MPE), F. Pacaud (Univ. Bonn), D. Eckert (Univ. Geneva), J. Sanders (MPE), N. Ota (Univ. Bonn), E. Bulbul (MPE), V. Ghirardini (MPE), MPE/IKI]](https://eoportal.org/ftp/satellite-missions/s/SpektrRG-SRG-020522/SpektrRG-SRG_Auto15.jpeg)
- “This is a dream come true. We now know that eROSITA can deliver on its promise and create a map of the whole X-ray sky with unprecedented depth and detail,” confirms Andrea Merloni, eROSITA Project Scientist. “The legacy value will be enormous. Beside the beautiful images like the ones we’re showing today, catalogues of millions of exotic celestial objects such as black holes, galaxy clusters, neutron stars, supernovae and active stars will be used by astronomers for years to come.”
- Launched on 13 July 2019 as part of the Russian-German Spektrum-Roentgen-Gamma (SRG) space mission, which also includes the Russian ART-XC telescope, eROSITA completed its 1.5 million kilometer journey to the second Lagrange point (L2) of the Earth-Sun-system on October 21, and has now – 100 days after launch – entered its target orbit around L2. The commissioning phase of the telescope was officially completed on October 13. While the scientific performance of the system is outstanding, this first phase was not problem-free.
- “The commissioning phase lasted longer than expected, after we found some anomalies in the electronic controls of the cameras,” explains Peter Predehl. “But teasing out these problems is exactly why we have such a phase. After a careful analysis we determined that the issues are not critical. We’re still working on them, but in the meantime the program can go forward normally.” The telescope has now entered the so-called calibration and performance verification (CalPV) phase, during which astronomical observations are carried out to better understand the instrument and verify its full potential to meet the scientific requirements. At the end of the CalPV phase, after a final review by the operations team, SRG and eROSITA will enter into its prime phase, the four year all-sky X-ray survey.
- The development and construction of the eROSITA X-ray telescope was led by the Max Planck Institute for Extraterrestrial Physics with contributions from the Institute for Astronomy and Astrophysics of the University Tübingen, the Leibniz Institute for Astrophysics Potsdam (AIP), University Observatory Hamburg, and Dr. Karl Remeis Observatory Bamberg, with the support of the German space agency DLR. The Ludwig-Maximilians-Universität Munich and the Argelander Institute for Astronomy of the University Bonn also participated in the science preparation for eROSITA. The Russian partner Institute is the Space Research Institute IKI in Moscow; NPOL, Lavochkin Association, in Khimky, near Moscow, is responsible for the technical implementation of the whole SRG mission, which is a joint project of the Russian and German space agencies, Roscosmos and DLR.
• The Spektr-RG observatory successfully separated from the upper stage! Next stage: 3 months after launch — cruise to L2, positioning, performance verification (PV) and calibration phase (Ref. 25).
- 4 years — all-sky survey in 0.3–11 keV energy band (Ref. 11);
- 2.5 years — observations of selected objects and regions in the harder X-ray range up to30 keV in a 3-axis stabilization mode.
Sensor complement: (eROSITA, ART-XC)
The scientific payload consists of two independent telescopes — a soft-X-ray survey instrument, eROSITA, being provided by Germany, and a medium-X-ray-energy survey instrument, ART-XC (Astronomical Roentgen Telescope- X-ray Concentrator), being developed by Russia.
eROSITA (extended ROentgen Survey with an Imaging Telescope Array):
eROSITA is an instrument designed and developed at the MPE (Max-Planck-Institut für Extraterrestrik), Garching, Germany (lead institute and project management) with DLR funding. Further cooperating institutions and companies in the eROSITA project are: Institut für Astronomie und Astrophysik der Universität Tübingen (IAAT); Astrophysikalisches Institut Potsdam (AIP); Dr.-Remeis-Sternwarte, Bamberg; Hamburger Sternwarte; Space Research Institute (IKI), Moscow; Roskosmos, Moscow; Kayser-Threde GmbH, Munich; Carl Zeiss AG, Oberkochen; MLT (Media Lario Technologies), Italy.
The general design of the eROSITA X-ray telescope is derived from that of ABRIXAS (A Broadband Imaging X-ray All-Sky Survey). A bundle of 7 mirror modules with short focal lengths make up a compact telescope which is ideal for survey observations. Similar designs had been proposed for the missions DUO (Dark Universe Observatory) and ROSITA but were not realized due to programmatic shortfall. Compared to those, however, the effective area in the soft X-ray band has now much increased by adding 27 additional outer mirror shells to the original 27 ones of each mirror module. The requirement on the on-axis resolution has also been confined, namely to 15 arc seconds HEW (Half Energy Width). For these reasons the prefix “extended” was added to the original name “ROSITA”. The scientific motivation for this extension is founded in the ambitious goal to detect about 100,000 clusters of galaxies which trace the large scale structure of the Universe in space and time. 44) 45)
The overall objectives of eROSITA are: 46) 47) 48) 49)
- An extension of the ROSAT imaging all-sky survey to higher X-ray energies up to 10 keV with unprecedented spectral and angular resolution. Thereby, eROSITA will act as a pathfinder for the next X-ray observatories, particularly the SIMBOL-X and the IXO (International X-ray Observatory) missions.
- With the detection of a sufficiently large number of clusters of galaxies, precise measurements will be feasible of the equation of state of Dark Energy and of “baryonic acoustic oscillations” in the power density spectrum of galaxy clusters.
The main scientific goals are: 50) 51) 52) 53) 54) 55) 56) 57)
• To detect systematically all obscured accreting Black Holes in nearby galaxies, as well as many (> 170000) new, distant active galactic nuclei
• To detect the hot intergalactic medium of 50-100 thousand galaxy clusters and groups and hot gas in filaments between clusters, so as to map out the large-scale structure in the Universe for the study of cosmic structure evolution
• To study in detail the physics of galactic X-ray source populations, like pre-main sequence stars, supernova remnants and X-ray binaries.
eROSITA will perform a deep survey of the entire X-ray sky. In the soft band (0.5-2 keV), it will be about 30 times more sensitive than ROSAT, while in the hard band (2-8 keV) it will provide the first ever true imaging survey of the sky. The design driving science is the detection of large samples of galaxy clusters to redshifts z > 1 in order to study the large scale structure in the Universe and test cosmological models including Dark Energy. In addition, eROSITA is expected to yield a sample of around 3 million AGN (Active Galactic Nuclei), including obscured objects, revolutionizing our view of the evolution of supermassive black holes and of their role in the structure formation process. The survey will also provide new insights into a wide range of astrophysical phenomena, including X-ray binaries, active stars and diffuse emission within the Galaxy.
The X-ray telescope of eROSITA consists of 7 identical and co-aligned mirror modules, each with 54 nested Wolter-1 type mirror shells. The mirror shells are glued onto a spider wheel which is screwed to the mirror interface structure making a rigid mechanical unit. The assembly of 7 modules forms a compact hexagonal configuration with 1300 mm diameter (Figure 31) and will be attached to the telescope structure which connects to the 7 separate CCD cameras in the focal planes. The co-alignment of the mirror module enables eROSITA to perform also pointed observations.

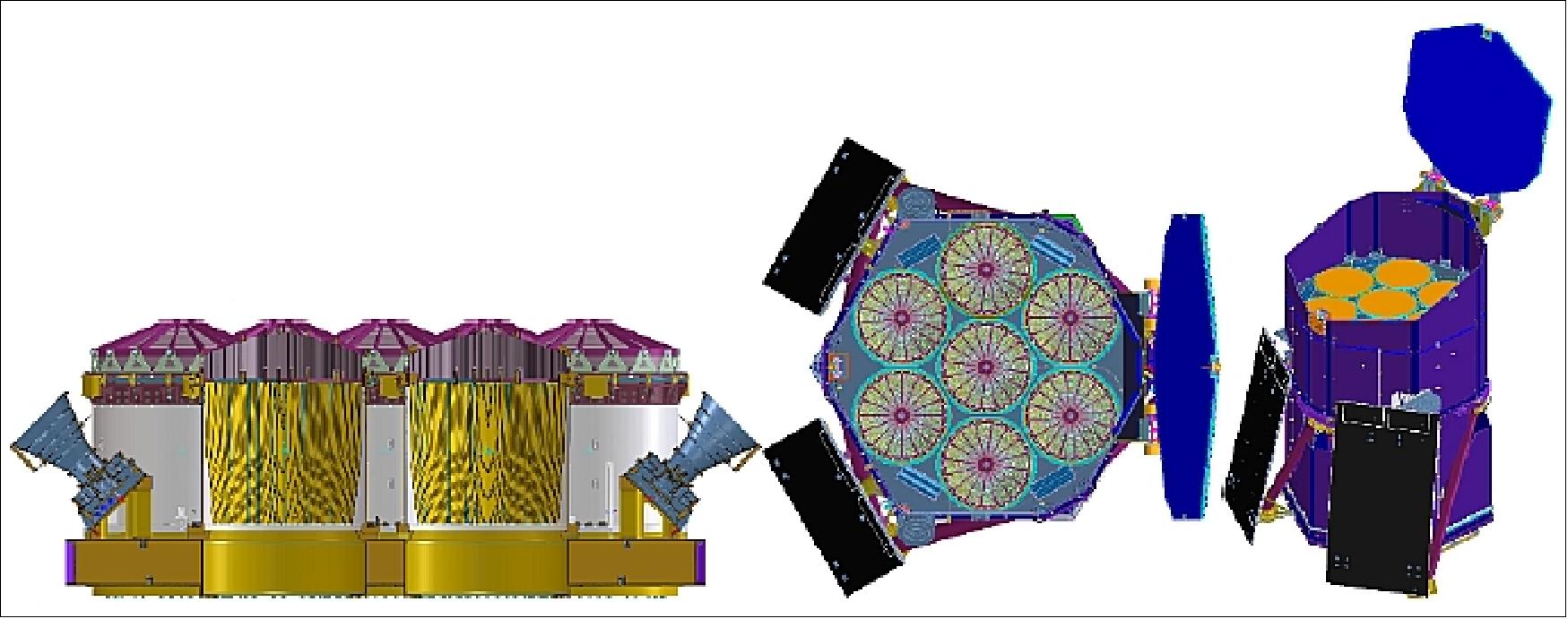
The mirrors manufacturing is based on a replication process from the ultra-smooth polished master. The mirror manufacturing process is divided into the following main steps (Figure 32):
1) The master is created in order to have a shape that is the negative of the final reflective surface of the mirror to be produced.
2) The master is then super-polished to have appropriate roughness and shape accuracy.
3) A layer of gold is deposited onto the master.
4) The master is then mounted on a support frame that holds it during electroforming, allowing also a proper rotation inside the galvanic bath. The master is then placed in the electroforming bath containing a proprietary chemistry, where the metal layer is deposited up to the desired thickness forming directly the mirror.
5) After the electroforming process is completed the mirror and the master are separated by thermal separation. The particular properties of the separation layer ensure a clean interface separation at the original master’s outer surface, thus reproducing the master’s optical surface quality onto the mirror.
6) Integration in VOB (Vertical Optical Bench).
7) X-ray test.
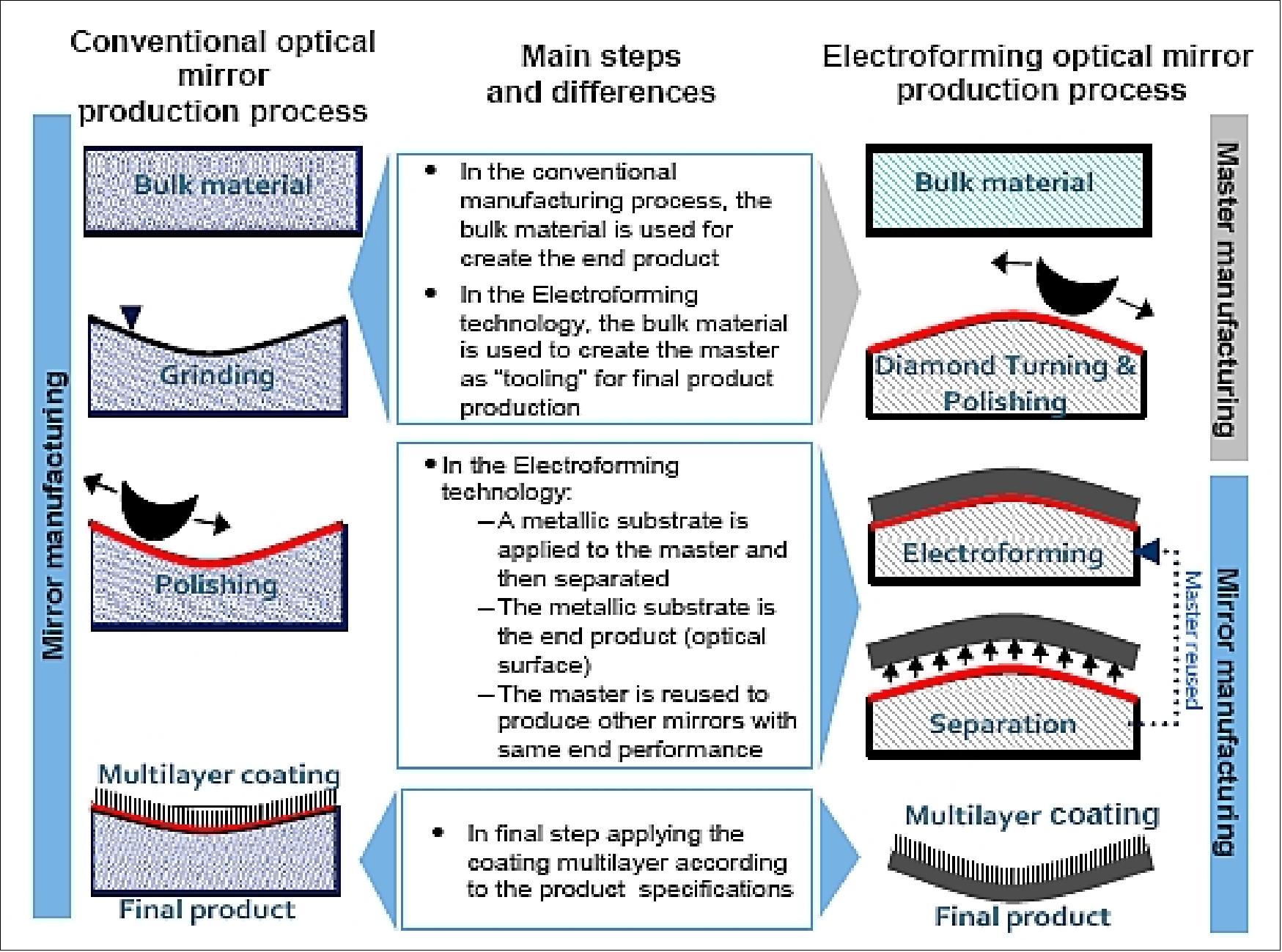
In the frame of the eROSITA mission Media Lario Technologies is in charge of the production of the new mandrels from n. 27 to n. 1, which are the bigger ones. The remaining mandrels from n. 54 to n. 28 are provided by the Max Planck Institute and are the ones used for the ABRIXAS mission (Ref. 44).
Mirror type | Wolter 1 |
Number of mirror modules | 7 |
Orientation of mirror modules | parallel |
Degree of nesting (mirror shells/module) | 54 |
Focal length | 1600 mm |
Largest mirror diameter | 358 mm |
Smallest mirror diameter | 76 mm |
Micro-roughness | < 0.5 nm |
Energy range | ~0.2 to 10 keV |
Energy resolution | 138 eV @ 6 keV |
Coating | Gold (> 50 nm) |
FOV (Filed of View) | 61 arcmin |
Telescope structure | ~ 1.9 m in diameter x 3.25 m in hight |
Total mass of the eROSITA instrument | ~ 750 kg, power = 405 W |
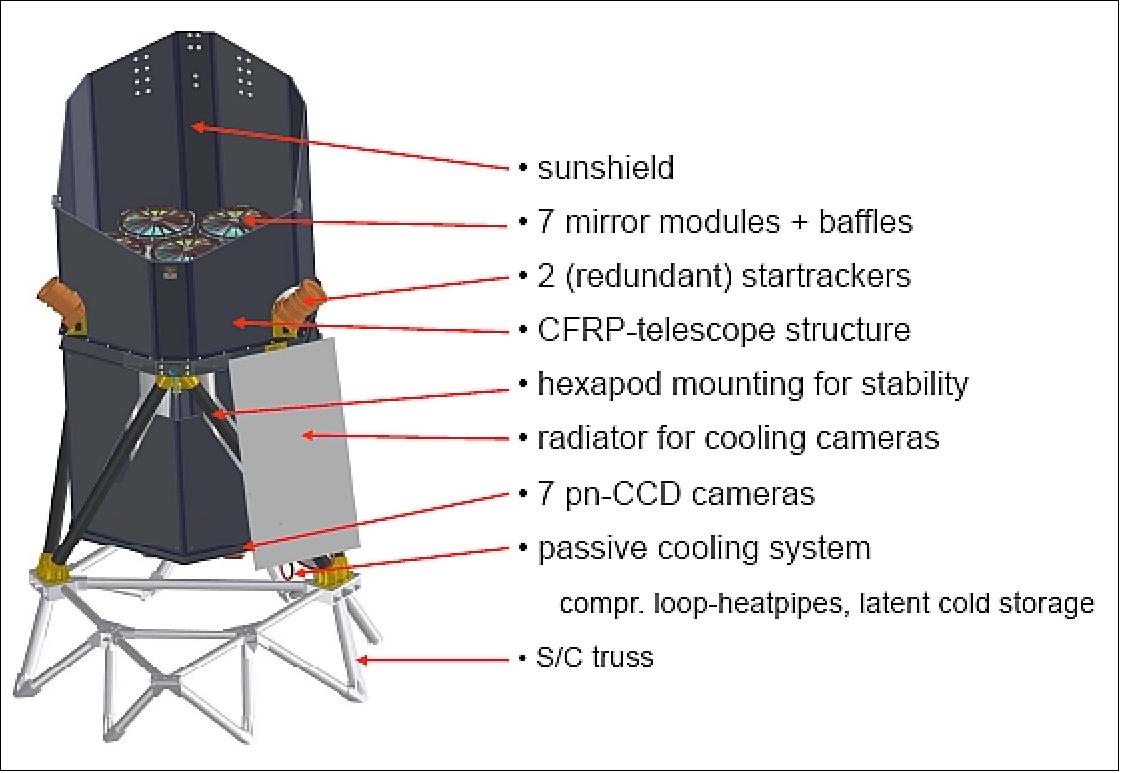
Each telescope consists of a highly nested mirror system with 54 paraboloid-hyperboloid mirror shells and a frame store pnCCD (pn-junction CCD detectors) camera with a 3 cm x 3 cm large image area (corresponding to a field of view of 1º in diameter on the satellite) with a pixel size of 75 µm by 75 µm. The imaging area consists of 384 x 384 pixels.
The camera is equipped with seven dedicated focal plane pnCCD detectors which are located at the focal point of each of the mirror modules. The pnCCD detector concept permits accurate spectroscopy of X-rays as well as imaging with high time resolution. The pnCCD detector is based on the successful XMM-Newton pnCCD detector concept but was further improved in terms of design and technology. 59)
In particular, a frame store section is added to the image area for the purpose of simultaneous imaging and a readout capability in separate CCD areas. The thickness of the whole pnCCD chip of 450 µm is uniformly sensitive to X-rays from very low up to very high energies. The X-ray photon detection efficiency is at least 90% in the energy band from 0.3 keV to 10 keV. Frame store operation allows very high frame rates of up to 200 X-ray images per second without smearing of the image (Figure 34).
Operating the CCD in frame store (i.e. frame transfer) mode is accomplished by transferring rapidly the image into the frame store area within 200 µs after the 50 ms exposure time. Since the frame store area is shielded against X-rays, the stored image can be read out without interference row by row, while the photons of the next image accumulate in the image area. The readout time is less than 10 ms for the complete image, in the remaining time the CAMEX (CMOS Amplifier and MultiplEXer) readout chip is switched into standby mode to minimize the average heat dissipation to the focal plane CCD.
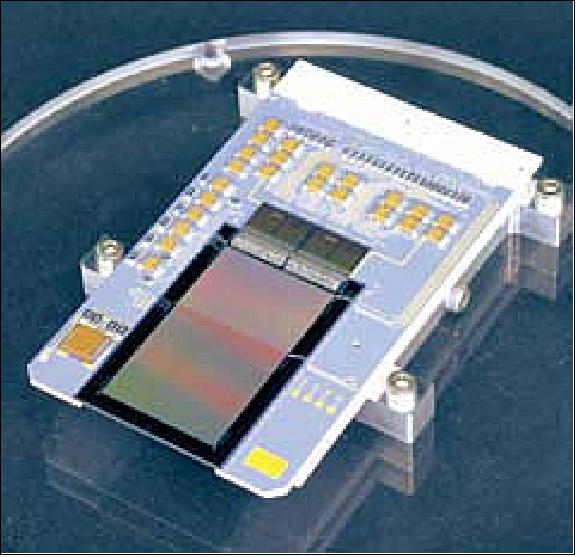
The pnCCD shows best energy resolution at temperatures below -60º C. For the satellite mission a lower temperature is required due to the proton environment which causes radiation damage by creating defects in the silicon lattice. The eROSITA pnCCD detector offers in addition the possibility to select the optimum gain out of a choice of 16 discrete levels. This option allows spectroscopy and imaging of higher energetic particles, e.g. electrons or protons, on ground as well as in space.
Detector module: The pnCCD is a column-parallel device. Each of the 384 CCD channels is equipped for this purpose with an anode, which is connected to the gate of the on-chip JFET (Junction Field-Effect Transistor). The CCD on-chip transistors are operated in source follower mode and are read out in parallel by the CAMEX chip. This mixed signal ASIC comprises 128 parallel readout channels; each of them includes an eightfold correlated double sampling filter. The specific settings, which determine one of the various possible operating modes, are stored in digital registers of the CAMEX. Programming is done via a serial interface. The ASIC features the following functional blocks:
- Current source to bias the pnCCD JFET
- JFET preamplifier for pnCCD signal amplification
- Programmable RC low pass for bandwidth limitation
- Eight-fold correlated double sampling filter
- Sample and hold stage
- 128 to 1 multiplexer
- Output buffer
- On-chip digital sequencer (16 x 64 bit)
- Serial programming interface with LVDS
- Option of integrated bias current DACs (digital analog converters).
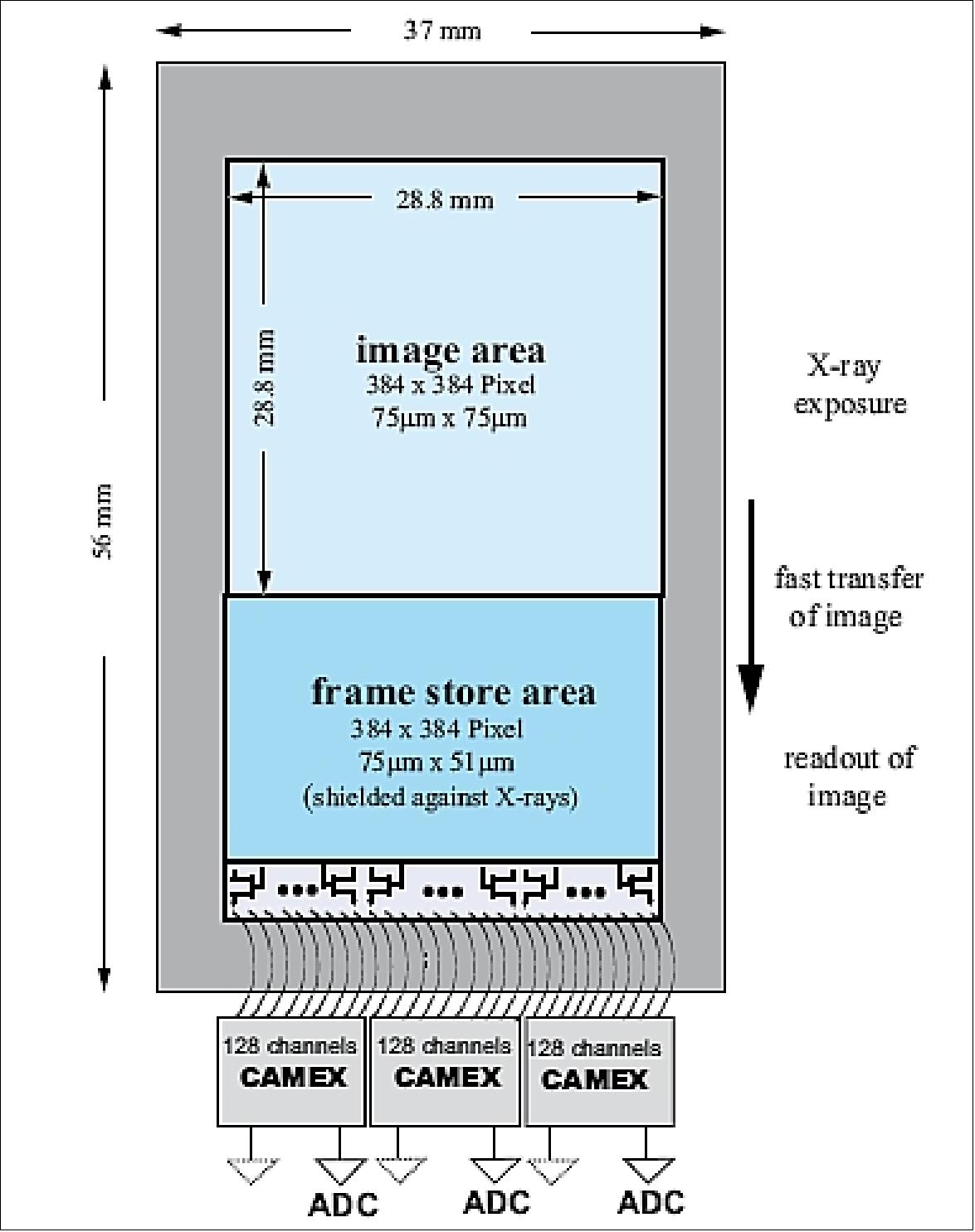
eROSITA camera: The entire eROSITA camera including control and readout electronics is developed in a close cooperation by MPE Garching and MPI-HLL (Max-Planck-Institut Halbleiterlabor), Munich.
The electronics of each eROSITA camera can be subdivided into frontend electronics nearby the detector and the other electronics, which is located in a particular box per camera system. That way the seven eROSITA cameras are independent of each other. Their arrangement is shown in Figure 36.
The camera housing provides also a thermal interface and protects the detector against light, dust and radiation (Figure 37). A cylindrical titanium block, which is glued on top of the Al2O3 detector PCB (Printed Circuit Board), is fitted into the CCD-module casing and fixed with a single screw (Figure 38). The aluminum CCD module casing is cooled to -80ºC using heat pipes; it is thermally insulated with MLI (Multilayer Insulation). The module is mounted with thin glass fiber brackets to the surrounding proton shield providing a temperature of approximately +20ºC.
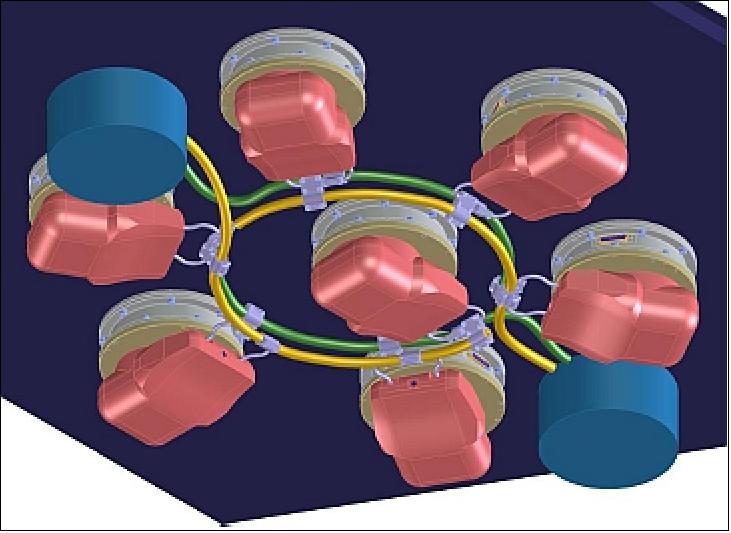
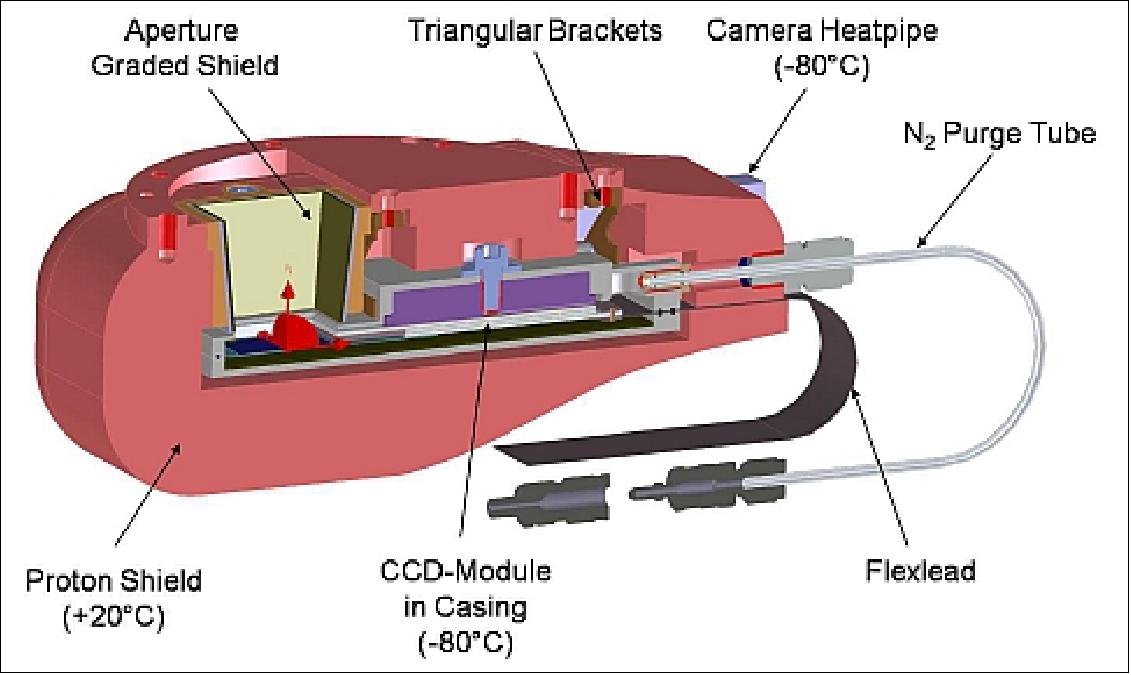
Legend to Figure 37: X-rays enter the pnCCD image area top left through the cut-out in the proton shield. While the copper proton shield is not cooled, the camera casing, which surrounds the CCD detector, is connected with a heat pipe to the central cooling system for all seven cameras. The electronics box with the DAQ (Data Acquisition) system belonging to the camera is not shown.
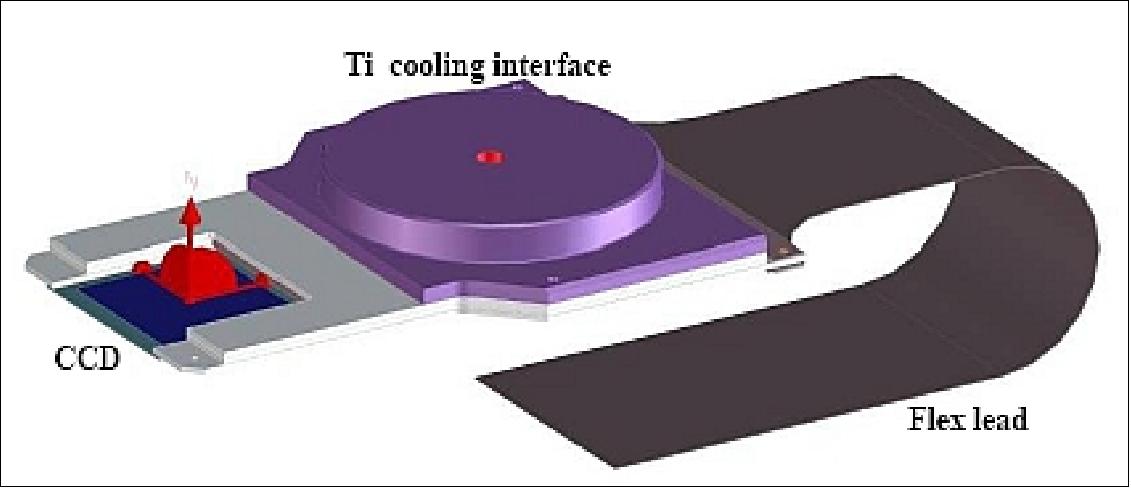
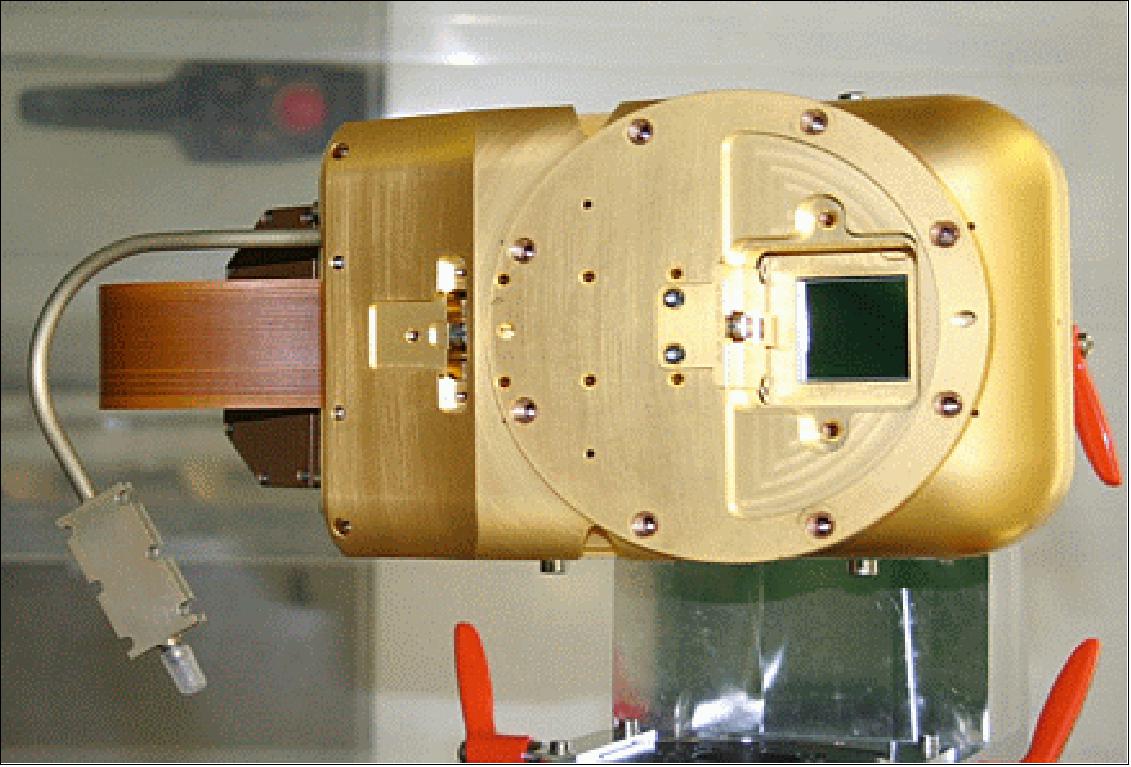
Data acquisition system electronics: Apart from the detector electronics, each camera comprises a DAQ system and electrical interfaces (Figure 40). The main interfaces are a synchronous bidirectional bus for telecommands and telemetry and a power line (+27 V nominal and redundant from power distribution unit).
The DAQ electronics provides the following subsystems:
- Power converters and control units. Some voltages are switchable and/or commandable by telecommands
- Sequencer with reprogrammable FPGA, which generates all necessary timing signals for the detector and the ADCs (Analog Digital Converters)
- ADCs, which digitize the differential analog CAMEX signals into 14 bit data for each CCD pixel per frame. The digital data are delivered to a DSP via a FIFO implemented in the FPGA using DMA transfers.
- DSP event processor: A digital signal processor (DSP) calculates offset as well as noise tables and is able to transmit unprocessed raw data for testing purposes. The DSP applies offset and common mode corrections, event thresholds (low and high), and optionally performs split event recognition. The processed data (with energy, position and time information) are transmitted to the interface controller. The event processor executes and distributes telecommands, collects housekeeping and science data and is able to upload new software to itself and the FPGA. Memory uploads can be stored temporarily in volatile memory or permanently in reprogrammable FLASH memory. The initial program will be stored in higher qualified boot-PROMs. The DSP uses its fast internal memory for calculations. An external memory is available for additional data tables and testing.
- Onboard calibration wheel control: Movement and position readout of the calibration mechanism with its selectable positions: open (nominal), closed (testing) and X-ray source (calibration).
The event processor must complete the digital signal processing of a frame, i.e. of 147,456 pixels, during the cycle time of 50 ms. This includes in particular for each pixel offset subtraction as well as common mode determination and subtraction per row. Finally, events are discriminated from noise by comparison with a low threshold and they are rejected, if a high threshold is exceeded, i.e. they are recognized as particles.
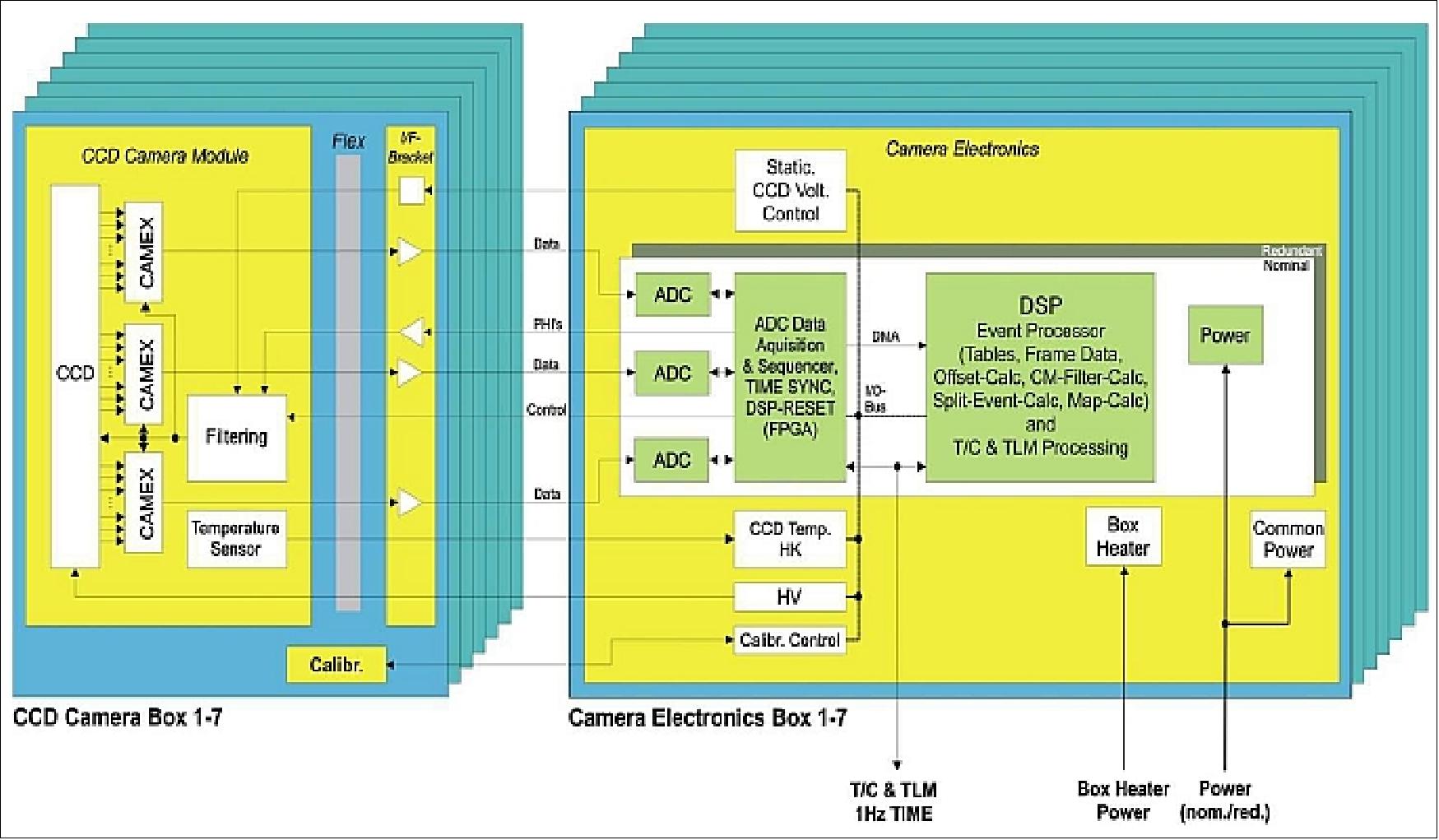
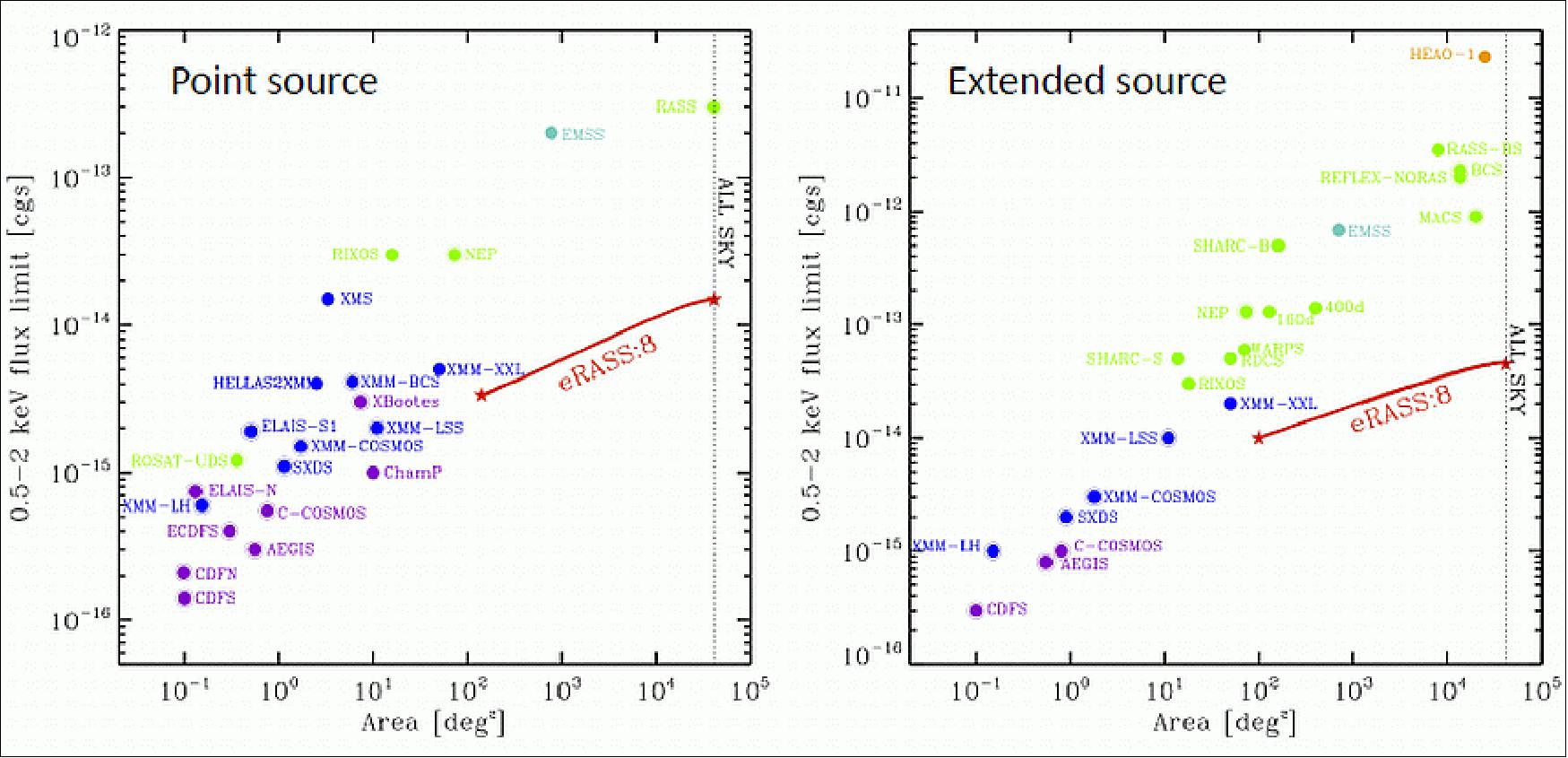
The point source sensitivity of eROSITA: ~30 times better than ROSAT (soft band 0.5-2 keV) and ~100 times better than HEAO/RXTE (hard band 2-10 keV).
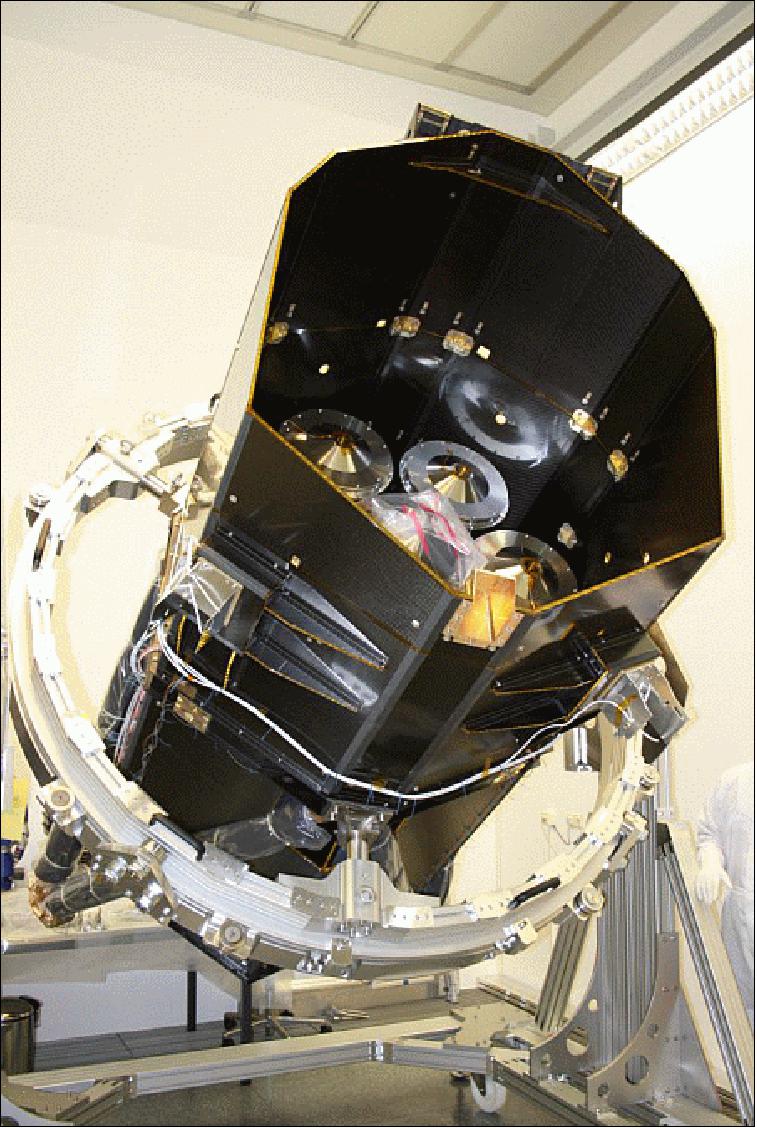
Data share policy of the SRG project:
Data Rights and Policies (MPE):
• German eROSITA data are made public after a 2 year proprietary period.
• Periodic data releases envisaged (e.g. 6, 24, 48 months)
• Proprietary data via eROSITA_DE collaboration (consortium)
• Projects/Papers regulated by Working Groups
• Individual External Collaborations
• Group External Collaborations.
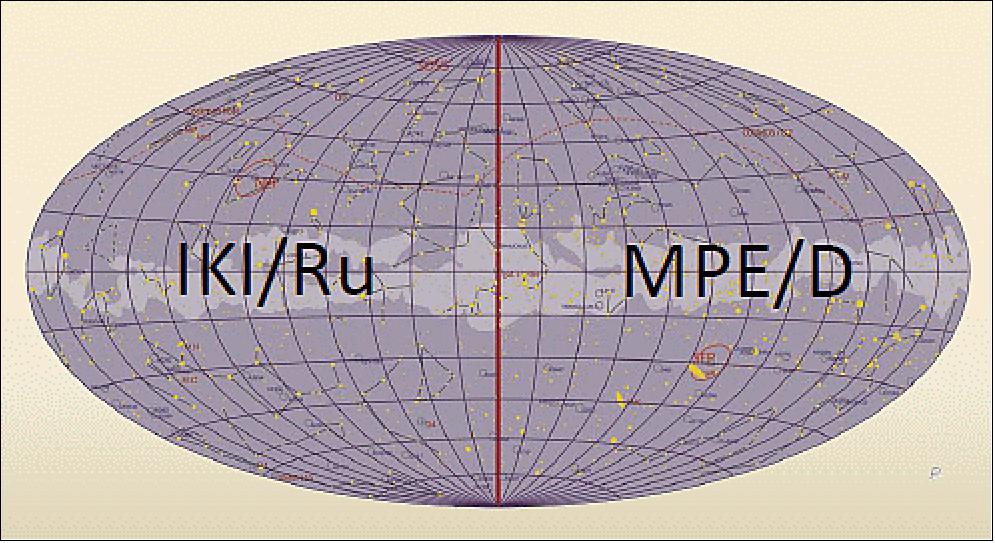
ART-XC (Astronomical Roentgen Telescope - X-ray Concentrator)
ART-XC is a Russian-led complementary instrument to eROSITA. It is also a 7-module X-ray telescope system that provides higher energy coverage, up to 30 keV (with limited sensitivity above 12 keV).
1) VNIIEF, Sarov – design of telescope, QM X-ray mirror systems, structure, tests, AIT 2) IKI, Moscow – CdTe DSSD X-ray detectors, aboard computer and memory, thermal balance system control block, star sensor, X-ray ground calibration of detectors and mirror systems 3) NASA/MSFC – FM X-ray mirror systems and their ground calibration 4) Lavochkin Association, Khimki – MLI, heat pipes, pyropin 5) Obninsk Research and Production Enterprise "Technologiya“ – carbon fiber structure |
ART-XC is an instrument of IKI-led (Space Research Institute), Moscow, Russia. The instrument is designed for the following tasks: 62) 63)
• All-sky X-ray survey in the 6-11 keV energy region with a sensitivity of 3 x 10-13 erg s-1 cm-2 keV-1; discovery in the course of survey at local Universe several thousand new AGNs (Active Galactic Nuclei)
• Study of intrinsically heavily absorbed/Compton thick AGNs (NH ≥ 3 x 1023 cm-2)
• Study of massive nearby galaxie clusters with T ≥ 4 keV in pointing observation mode
• Study heavily obscured galactic X-ray binary systems
• Study broadband spectra of Galactic objects (including binary systems, anomalous pulsars, supernova remnants) up to30 keV, spectroscopy and timing of point sources
• Study non-thermal component in the Galaxy diffuse emission
• Search for cyclotron line features X-ray pulsar spectra.
Instrument: ART-XC will consist of seven independent, but co-aligned, telescope modules with seven corresponding cadmium telluride (CdTe) focal plane detectors. Each will operate over the approximate energy range of 6-30 keV, with an angular resolution of 1 arcmin, a field of view of ~30 arcmin and an energy resolution about 10% at 14 keV. NASA/MSFC (Marshall Space Flight Center) fabricated 4 of the 7 mirror modules, to complement those fabricated by VNIIEF (All-Russian Federal Nuclear Center) in Russia. 64) 65) 66) 67)
Parameter | ART-XC | eROSITA |
Energy range | 5-30 keV | 0.2-12 keV |
Effective area | 455 cm2 at 8 keV | 2500 cm2 at 1 keV |
FOV (Filed of View) | 34 arcmin | 1º |
System angular resolution (on axis) | ≤1 arcmin | 15 arcsec |
Energy resolution | 1.4 keV at 14 keV | 130 eV at 6 keV |
ART-XC is the smaller one of the two telescopes and has a worse resolution compared to eROSITA. But it works in the range of 5-30 keV and therefore is used for the higher-energetic X-rays. The combination of both telescopes will result in an extremely detailed broadband all sky survey.
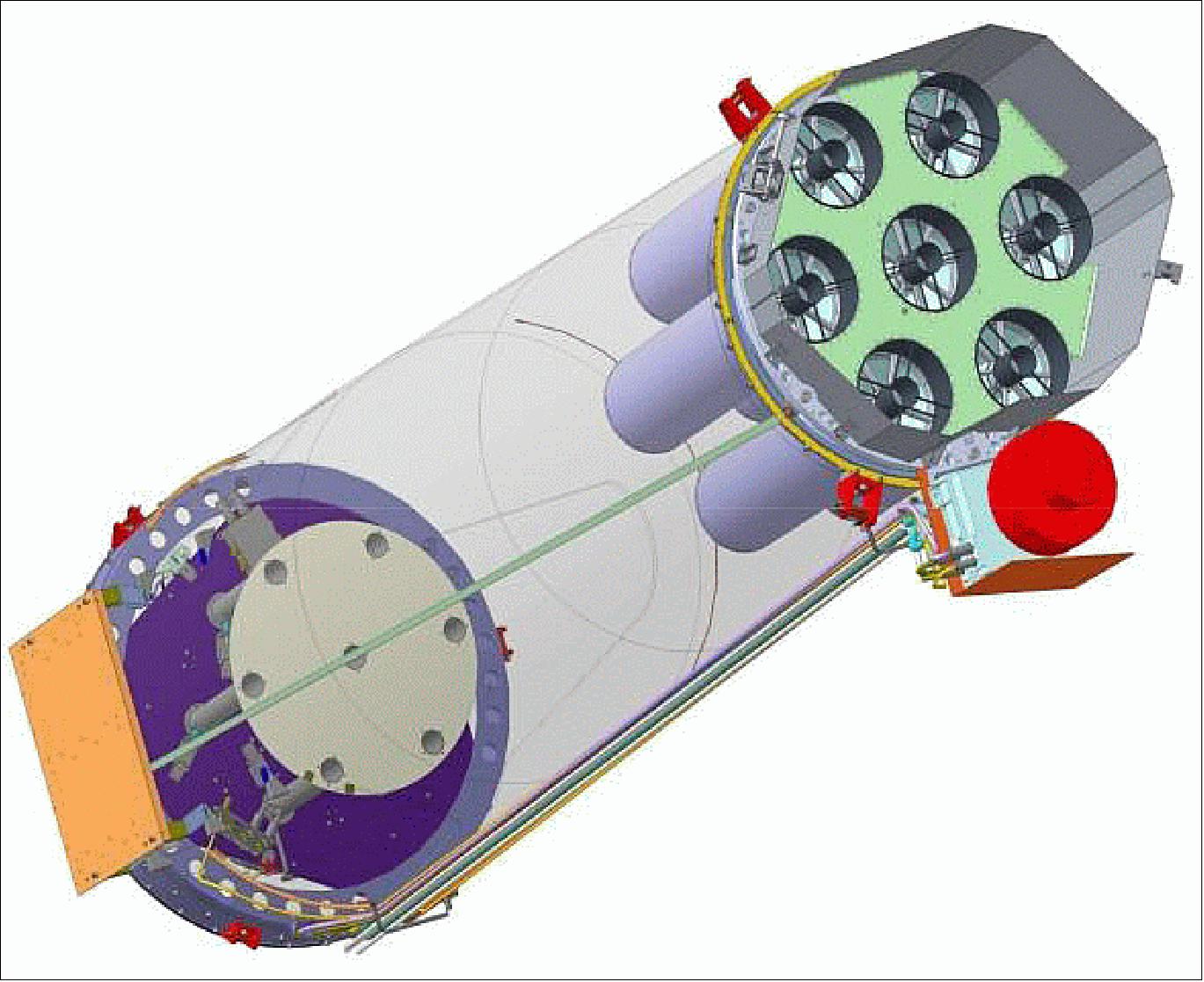
Telescope consist of 7 identical co-aligned mirror modules, each nesting 28 mirror shells. Shells were fabricated at the Marshall Space Flight Center and made from nickel-cobalt alloy with iridium coating. The telescope modules have a large field of view (0.3º x 0.3º, 36' diameter), good angular resolution (HPD~ 30” on-axis at 8 keV) and wide energy range from 5 to 30 keV.

Number of mirror systems | 7 |
Number of nested mirror shells | 28 |
Mirror shells and coating materials | Nickel and Iridium |
Focal length | 2700 mm |
FOV | ∅34 arcmin |
On-axis resolution | ≤ 1 arcmin |
Effective area for pointed observations | 510 cm2 @ 7 keV |
Grasp for survey | 45º2 cm2 @ 7 keV |
Detector type | CdTe Schottky Diode double sided strip (ACRORAD) |
Crystal size | 30 mm x 30 mm x 1 mm |
No of strips | 41 x 41 |
Strip width | 550 µm |
Inter-strip distance | 75 µm |
ASIC | VA64TA |
Energy range | 5-30 keV |
Energy resolution | 10% at 14 keV |
Time resolution | 1 ms |
Be window thickness | 100 µm |
Power consumption | 300 W |
Total instrument mass | 350 kg |
X-ray optics:
• The X-ray modules are fabricated by the VNIIEF (Russia) and MSFC (USA)
• The NASA-IKI reimbursable agreement has been signed on February 7, 2011 to build 4 flight units
• NASA is to deliver 4 flight modules for the ART-XC instruments by the summer of 2014
• The work at the MSFC has been started on March 24, 2011.
X-ray optics designs:
• Both the VNIIEF and the MSFC designs are based on the single spider scheme
• The shell diameters vary from 50 to 150 mm
• The VNIIEF X-ray module design calls for the mirror shell thickness of 250 µm. The vibration tests for the qualification unit are in progress
• MSFC is exploring the variable thickness option. The design of the ART spider and housing is in progress.
Finding the traces of black holes requires X-ray vision. But unlike visible light, X-rays only reflect off surfaces at glancing angles - as a rock might skip off the surface of a lake. X-ray mirrors must therefore be designed with the reflecting surfaces almost parallel to the incoming X-rays. Just one mirror isn't enough, however, to collect very much X-ray light. To intercept as much light as possible, X-ray telescopes require a series of nearly parallel mirror surfaces. This is accomplished with the use of thin shells of mirrors, nested together like Russian dolls. 68)
- The challenge for ART-XC's mirrors is that high-energy X-ray light reflects at angles that are even more glancing, or close to parallel. As a result, more shells are needed. To collect enough X-ray light, ART-XC has seven sets of 28 nested shells, with special reflective coatings. Each mirror is most efficient at reflecting a particular range of X-ray wavelengths.
- ART-XC's mirrors are electroformed from a nickel-cobalt alloy and coated with ultra-thin layers of reflective iridium coating which are smooth to 0.5 nanometers - about 1/1000 the wavelength of visible light. Researchers at Marshall have been developing and refining this mirror-making technology since the 1990s. SRG provides the first opportunity to use it on an orbiting X-ray observatory.
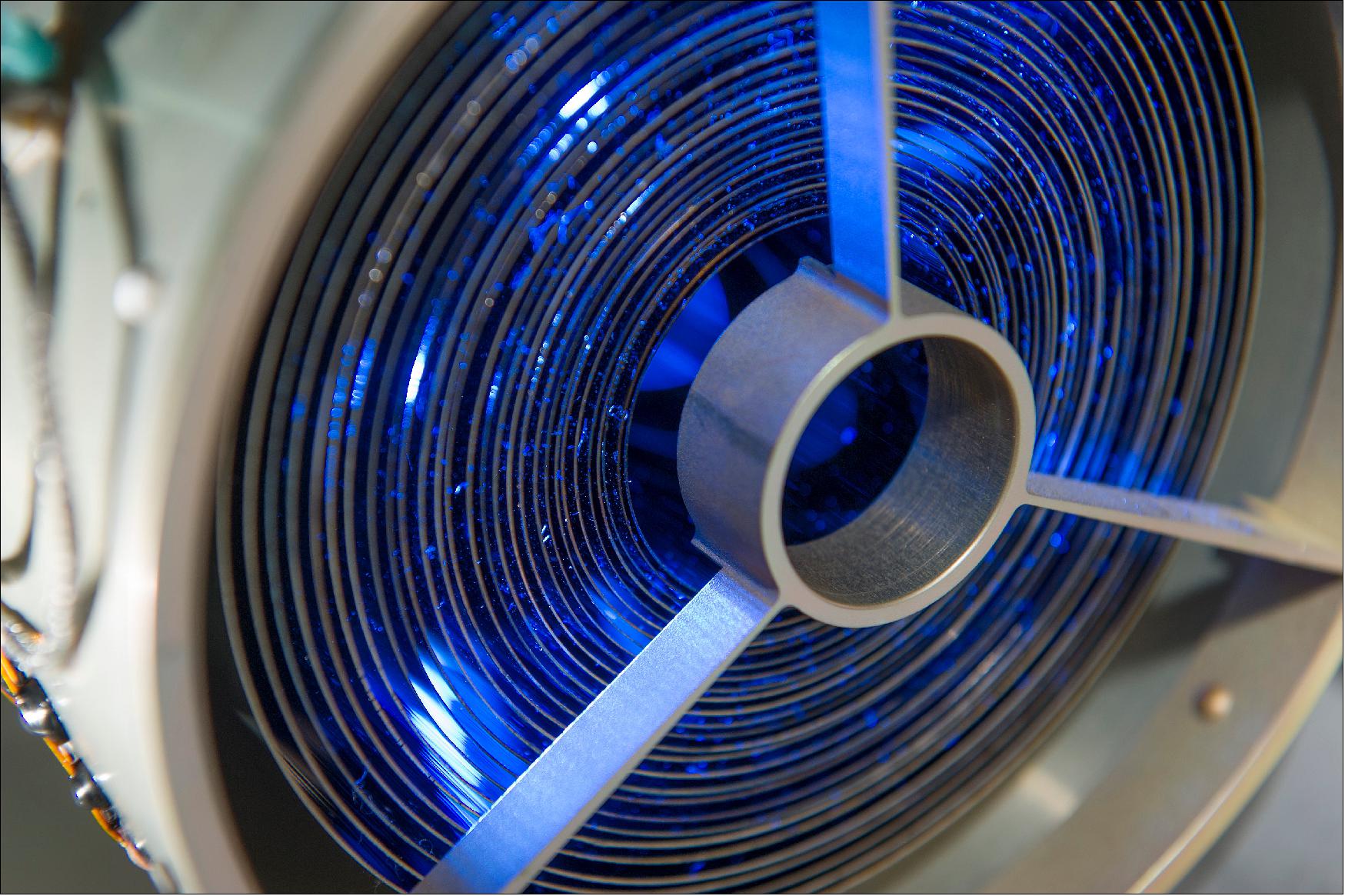
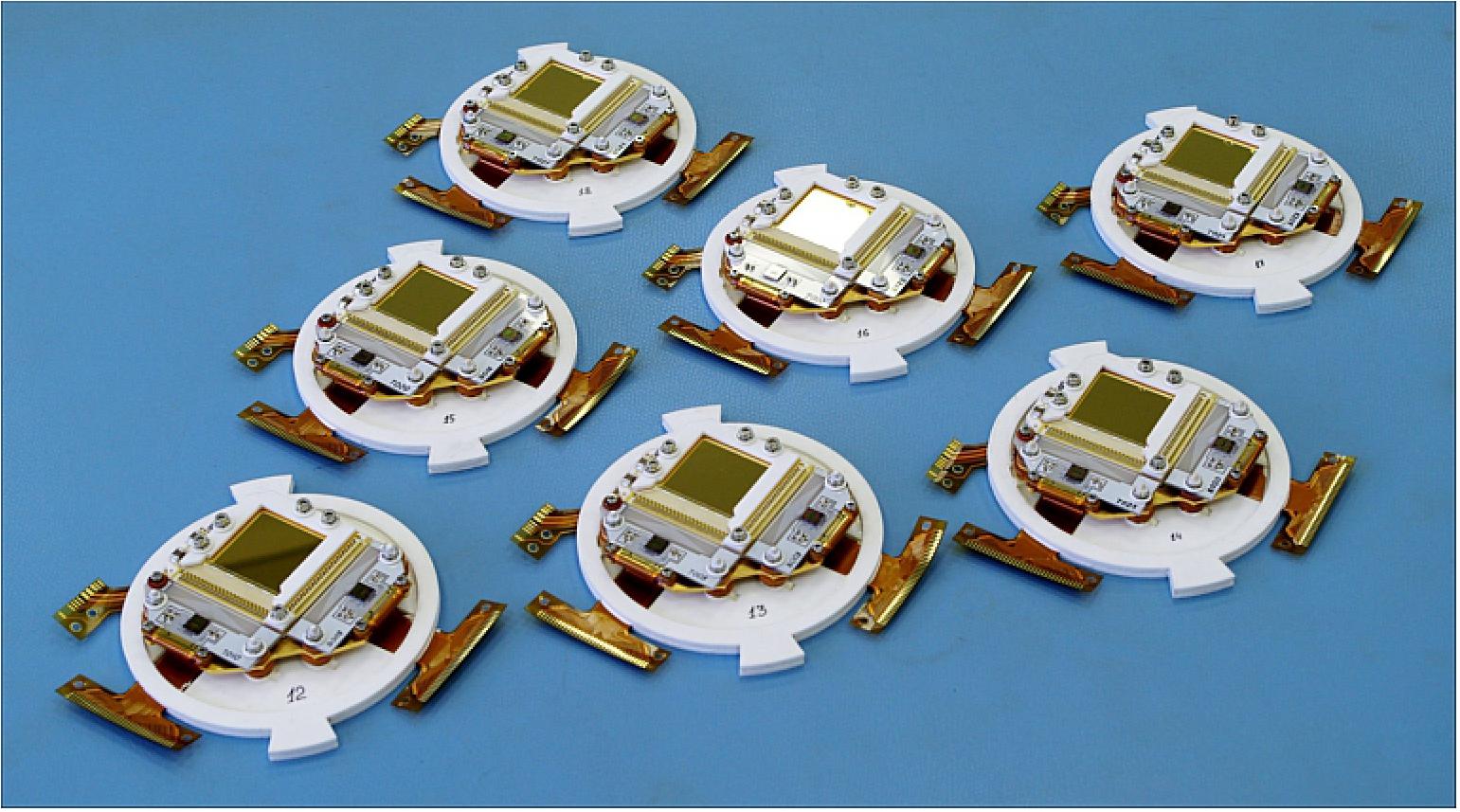
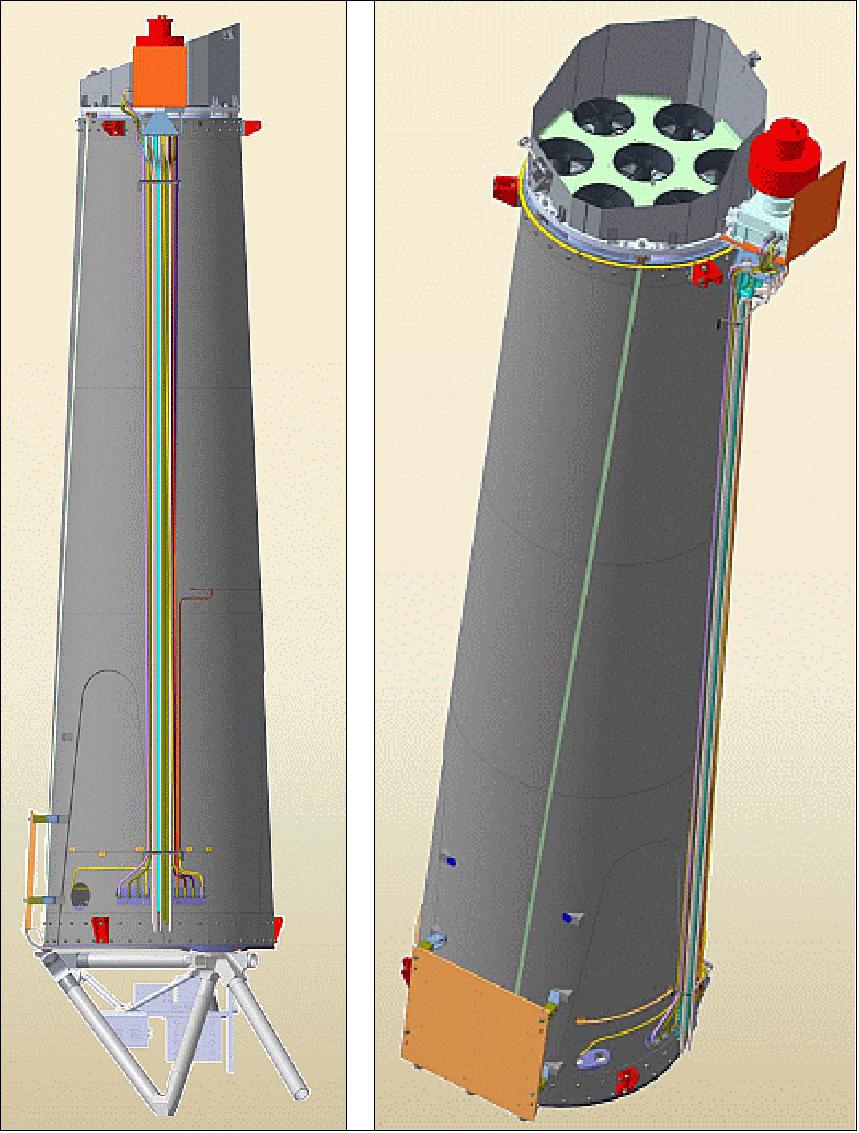
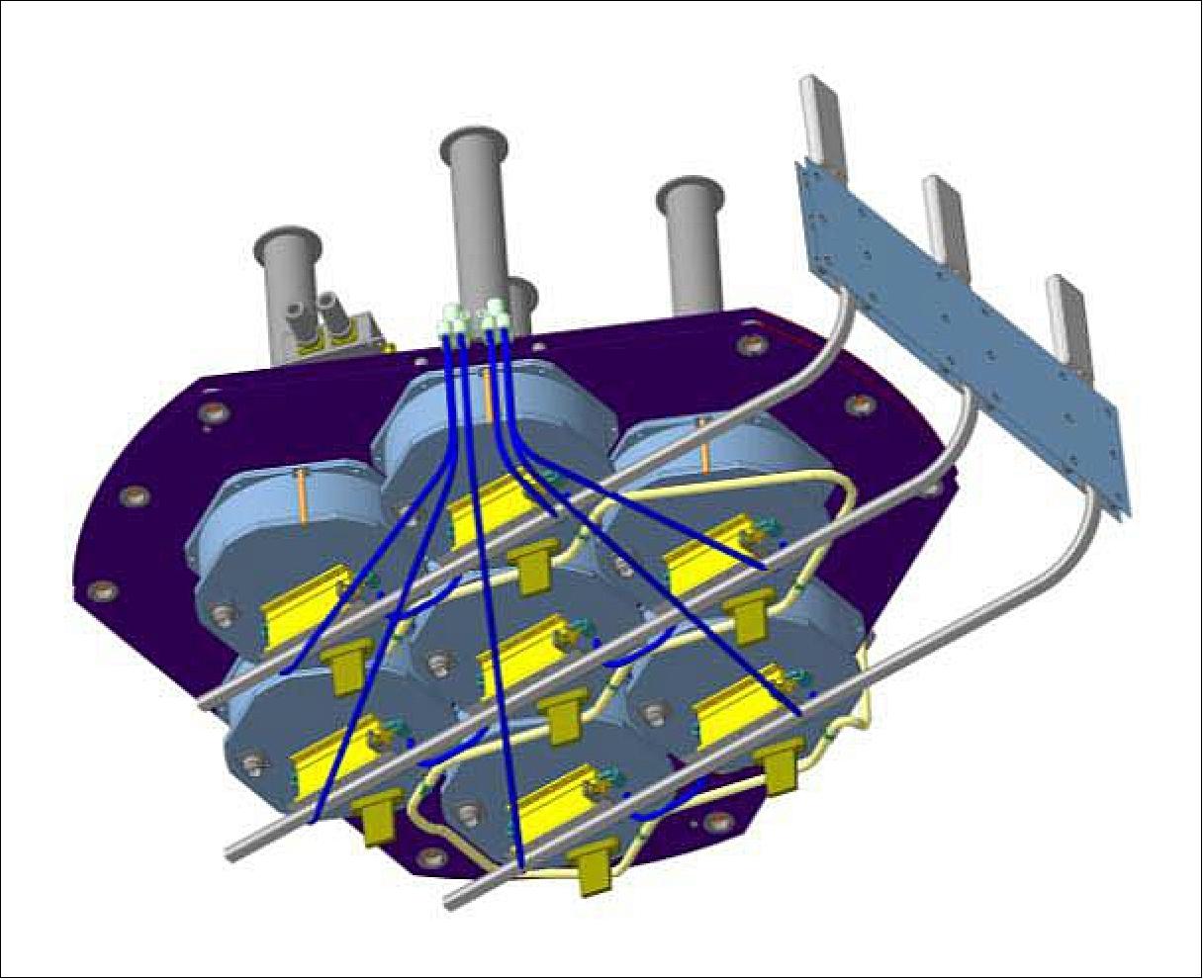
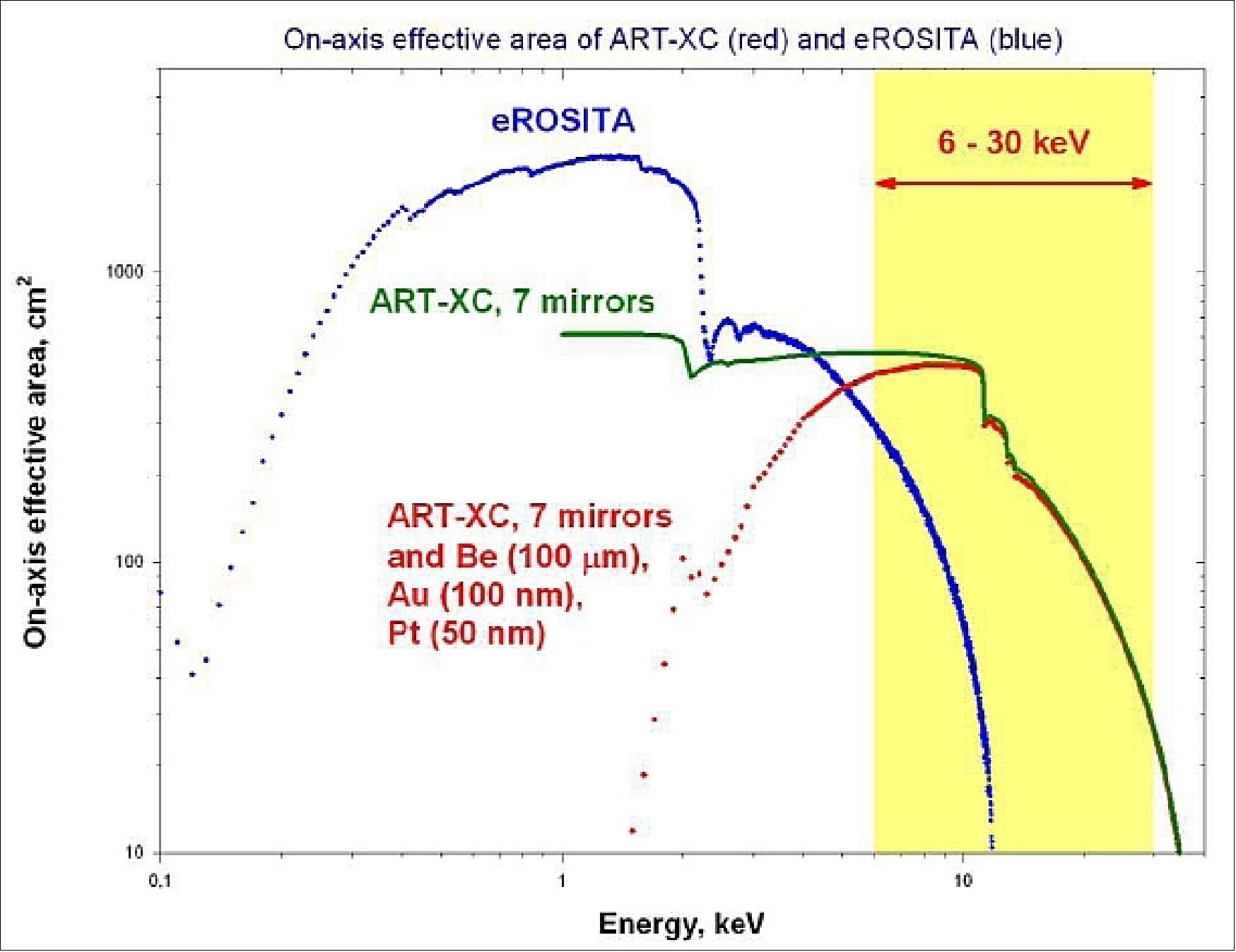
1) “eROSITA X-ray telescope: DLR and Roskosmos sign agreement in Moscow,” Aug. 18, 2009, URL: http://www.dlr.de/en/desktopdefault.aspx/tabid-5105/8598_read-19140/
2) A. Merloni, P. Predehl, W. Becker, H. Böhringer, T. Boller, H. Brunner, M. Brusa, K. Dennerl, M. Freyberg, P. Friedrich, A. Georgakakis, F. Haberl, G. Hasinger, N. Meidinger, J. Mohr, K. Nandra, A. Rau, T. H. Reiprich, J. Robrade, M. Salvato, A. Santangelo, M. Sasaki, A. Schwope, J. Wilms, and the German eROSITA Consortium, Edited by S. Allen, G. Hasinger, K. Nandra, “eROSITA Science Book: Mapping the Structure of the Energetic Universe,” Sept. 2012, arXiv:1209.3114 [astro-ph.HE], URL: http://www.aip.de/en/research/research-area-ea/research-groups-and-projects/galaxies/x-ray/erosita/erosita_science_book.pdf
3) “Spectrum-RG/eROSITA/Lobster Mission Definition Document,” Sept. 2005, URL: https://web.archive.org/web/20220704155522/http://hea.iki.rssi.ru/SXG/PROJECT/SXG-eng.htm
4) https://web.archive.org/web/20180705032827/http://hea.iki.rssi.ru/SRG/en/index.php
5) M .Pavlinsky, R .Sunyaev, E. Churazov, M. Gilfanov, A. Vikhlinin, G. Hasinger, P. Predehl, K. Mitsuda, R. Kelley, D. McCammon, T. Ohashi, J. W. den Herder, B. Ramsey, M. Gubarev, S. O’Dell, R. Fujimoto, “Spectrum-Roentgen-Gamma astrophysical mission,” 'Space Telescopes and Instrumentation 2008: Ultraviolet to Gamma Ray,' edited by Martin J. L. Turner, Kathryn A. Flanagan, Proceedings of SPIE, Vol. 7011, 70110H, (2008), Marseille, France, July 2008
6) Peter Predehl on behalf of the eROSITA team, “eROSITA,” eROSITA, Area-F Kickoff Meeting, Garching 2007, URL: http://www.universe-cluster.de/MaKaC/getFile.py/access?contribId=13&resId=0&materialId=slides&confId=245
7) N. Cappelluti, P. Predehl, H. Böhringer, H. Brunner, M. Brusa, V. Burwitz, E. Churazov, K. Dennerl, A. Finoguenov, M. Freyberg, P. Friedrich, G. Hasinger, E. Kenziorra, I. Kreykenbohm, G. Lamer, N. Meidinger, M. Mühlegger, M. Pavlinsky, J. Robrade, A. Santangelo, J. Schmitt, A. Schwope, M. Steinmitz, L. Strüder, R. Sunyaev, C. Tenzer, “eROSITA on SRG,” Memorie della SAIt (Societa Astronomica Italinana), Vol. 1.1, 2008
8) G. W. Fraser, “The Spectrum Roentgen-Gamma (SRG) Mission and the Lobster Eye Wide Field Telescope (LWFT),” LWFT Science Meeting, University of Leicester, May 11, 2007, URL: http://www.star.le.ac.uk/lwft/presentations/fraser.pdf
9) http://www2.le.ac.uk/departments/physics/research/src/Missions/magex/public/LXO-Hardware.ppt/at_download/file
10) “Spectrum-RG/eROSITA/Lobster Mission Definition Document,” September 2005, URL: https://www.mpe.mpg.de/462254/MDD-6.pdf
11) Spektr-RG booklet, English version, 2019, URL: https://web.archive.org/web/20211120065654/http://hea.iki.rssi.ru/PDF/srg_2019_eng.pdf
12) Mikhail Pavlinsky on behalf of the SRG team, “Spectrum-Roentgen-Gammaastrophysical project,current status,” AXRO (Astronomical X-Ray Optics) 2009, Prague, Czech Republic, December 7, 2009
13) M. Pavlinsky; R. Sunyaev; E. Churazov; A. Vikhlinin; S. Sazonov; M. Revnivtsev; V. Arefiev; I. Lapshov; V. Akimov; V. Levin; M. Buntov; N. Semena; S. Grigorovich; V. Babyshkin; P. Predehl; G. Hasinger; H. Böhringer; J. Schmitt; A. Santangelo; A. Schwope; J. Wilms, “Spectrum-RG astrophysical project,” Proceedings of SPIE, Vol. 7437, 743708 (2009); doi:10.1117/12.837361
14) URL: http://www.russianspaceweb.com/spektr_rg.html
15) Peter Predehl on behalf of the SRG Collaboration, “Spektr-Rentgen-Gamma,” The X-ray Universe 2014 Symposium, Dublin, Ireland, June 16-19, 2014, URL: http://xmm.esac.esa.int/external/xmm_science/workshops/2014symposium//presentations/PPredehl_t.pdf
16) ”The entire sky in X-rays,” MGP (Max Planck Gesellschaft), 22 June 2019, URL: https://www.mpg.de/13559111/erosita
17) ”eROSITA arrives at Baikonur,” MPE Press Release, 26 April 2019, URL: http://www.mpe.mpg.de/7187100/news20190426
18) Anatoly Zak, ”Spektr-RG makes progress toward launch,” Russian Spaceweb, 18 September 2018, URL: http://www.russianspaceweb.com/spektr-rg-2018.html
19) ”A journey of a million miles begins with a single step: eROSITA travels to Russia for launch into deep space in 2018,” MPE, January 20, 2017, URL: http://www.mpe.mpg.de/6686210/news-20170120
20) ”11/2016 Project News: eROSITA leaves MPE,” eROSITA News, URL: http://www.mpe.mpg.de/450698/news
21) The eROSITA Bulletin, No 7, April 2016, URL: http://www.mpe.mpg.de/6541082/eROSITA_Bulletin7.pdf
22) Anatoly Zak, ”Proton sends Spektr-RG into deep space,” Russian SpaceWeb, 13 July 2019, URL: http://www.russianspaceweb.com/spektr-rg-launch-attempt2.html
23) Stephen Clark, ”Russia ships X-ray astronomy satellite to launch site,” Spaceflight Now, 25 April 2019, URL: https://spaceflightnow.com/2019/04/25/russia-ships-x-ray-astronomy-satellite-to-launch-site/
24) https://sputniknews.com/science/201812091070523644-russia-germany-space-observatory-launch/
25) ”Spektr-RG launch successful,” IKI, 15 July 2019, URL: [web source no longer available]
26) N. Eismont, A. Ledkov, R. Nazirov, D. Dunham, E. Chumachenko, “Optimal Transfer to Solar – Terrestrial Collinear Libration Points,” Proceedings of SpaceOps 2012, The 12th International Conference on Space Operations, Stockholm, Sweden, June 11-15, 2012
27) ”Statement on the status of the eROSITA instrument aboard Spektr-RG (SRG),” MPE, 02 March 2022, URL: https://www.mpe.mpg.de/7856215/news20220303
28) ”MPE shares institutional Marcel Grossmann Award for development of eROSITA aboard SRG,” MPE, 05 July 2021, URL: https://www.mpe.mpg.de/7719713/news20210705
29) ”The eROSITA X-ray sky in 360º,” MPE News, 17 May 2021, URL: https://www.mpe.mpg.de/7585671/news20210420?c=260780
30) ”Hoinga: The largest supernova remnant ever discovered with X-rays,” MPE, 03 March 2021, URL: https://www.mpe.mpg.de/7572057/news20210303?c=260760
31) W. Becker, N. Hurley-Walker, Ch. Weinberger, L. Nicastro, M. Mayer, A. Merloni, J. Sanders, ”Hoinga: A supernova remnant discovered in the SRG/eROSITA All-Sky Survey eRASS1,” Astronomy & Astrophysics, Published: 22 February 2021, https://doi.org/10.1051/0004-6361/202040156, URL: https://www.aanda.org/articles/aa/pdf/forth/aa40156-20.pdf
32) Notes:
1) The name Hoinga for the supernova remnant was chosen in honor of the first author’s hometown: Hoinga was the medieval name of Bad Hönningen am Rhein.
2) On 11 June 2020, the eROSITA telescope completed its first survey of the entire X-ray sky. Launched on 13 July 2019 on-board the SRG spacecraft and now orbiting the second Lagrange point of the Earth-Sun-system, the telescope is in continuous scanning mode. eROSITA is the primary instrument aboard SRG, a joint Russian-German science mission supported by the Russian Space Agency (Roskosmos), in the interests of the Russian Academy of Sciences represented by its Space Research Institute (IKI), and the Deutsches Zentrum für Luft- und Raumfahrt (DLR). The development and construction of the eROSITA X-ray instrument was led by the Max Planck Institute for Extraterrestrial Physics (MPE), with contributions from the Dr. Karl Remeis Observatory Bamberg, the University of Hamburg Observatory, the Leibniz Institute for Astrophysics Potsdam (AIP), and the Institute for Astronomy and Astrophysics of the University of Tübingen, with the support of DLR and the Max Planck Society. The Argelander Institute for Astronomy of the University of Bonn and the Ludwig-Maximilians-Universität Munich also participated in the science preparation for eROSITA.
33) ”eROSITA finds large-scale bubbles in the halo of the Milky Way,” MPE, 9 December 2020, URL: https://www.mpe.mpg.de/7540556/news20201210?c=450698
34) Peter Predehl, R. A. Sunyaev, W. Becker, H. Brunner, R. Burenin, A. Bykov, A. Cherepashchuk, N. Chugai, E. Churazov, V. Doroshenko, N. Eismont, M. Freyberg, M. Gilfanov, F. Haberl, I. Khabibullin, R. Krivonos, C. Maitra, P. Medvedev, A. Merloni, K. Nandra, V. Nazarov, M. Pavlinsky, G. Ponti, J. S. Sanders, M. Sasaki, S. Sazonov, A. W. Strong, J. Wilms, ”Detection of large-scale X-ray bubbles in the Milky Way halo,” Nature, Vol. 588, pp: 227-231, https://doi.org/10.1038/s41586-020-2979-0, Published: 9 December, 2020
35) ”ESA listens in on black hole mission,” ESA / Enabling & Support / Operations, 24 June 2020, URL: http://www.esa.int/Enabling_Support/Operations/ESA_listens_in_on_black_hole_mission
36) ”eROSITA takes its first look at the hot Universe,” DLR News, 22 October 2019, URL: https://www.dlr.de/content/en/articles/news/2019/04/20191022_first-light-erosita.html
37) ”Our deepest view of the X-ray sky,” MPE Press Release, 19 June 2020, URL: http://www.mpe.mpg.de/7461761/news20200619
38) ”Presskit for the eROSITA First All-Sky Survey,” MPE Press Kit, 19 June 2020, URL: http://www.mpe.mpg.de/7461950/erass1-presskit
39) ”Russian-German Telescope Completes First All-Sky Hard X-Ray Survey,” Sputnik News, 11 June 2020, URL: https://sputniknews.com/science/202006111079579803-russian-german-telescope-completes-first-all-sky-hard-x-ray-survey/
40) Hannelore Hämmerle, ”First sighting of hot gas sloshing in galaxy cluster,” MPE, 13 January 2020, URL: http://www.mpe.mpg.de/7399061/news20200113
41) J. S. Sanders, K. Dennerl, H. R. Russell, D. Eckert, C. Pinto, A. C. Fabian, S. A. Walker, T. Tamura, J. ZuHone and F. Hofmann, ”Measuring bulk flows of the intracluster medium in the Perseus and Coma galaxy clusters using XMM-Newton,” Astronomy & Astrophysics, Volume 633, Published online: 10 January 2020, https://doi.org/10.1051/0004-6361/201936468
42) ”With the start of the SRG all-sky survey, eROSITA promises most accurate maps of the X-ray sky ever,” MPE News, 8 December 2019, URL: http://www.mpe.mpg.de/7388144/news20191208?c=260780
43) ”Revealing the Beauty of the Hidden Universe: eROSITA sees first light,” MPE, 22 October 2019, URL: http://www.mpe.mpg.de/7362095/news20191022
44) L. Arcangeli, G. Borghi, H. Bräuninger, O. Citterio, I. Ferrario, P. Friedrich, G. Grisoni, F. Marioni, P. Predehl, M. Rossi, A. Ritucci, G. Valsecchi, D. Vernani, “The eROSITA X-ray Mirrors - Technology and Qualification Aspects of the Production of Mandrels, Shells and Mirror Modules,” ICSO 2010 (International Conference on Space Optics), Rhodes Island, Greece, Oct. 4-8, 2010, URL: http://congrex.nl/ICSO/Papers/Session%2013a/FCXNL-10a02-1987661-1-Ferrario_icso_paper.pdf
45) Vadim Burwitz for the eROSITA Team, “eROSITA,” 2010, URL: http://www.iachec.org/meetings/2010/Presentations/Burwitz_eROSITA_IACHEC_2010.pdf
46) Peter Predehl, Hans Böhringer, Hermann Brunner, Marcella Brusa, Vadim Burwitz, Nico Cappelluti, Evgeniy Churazov, Konrad Dennerl, Michael Freyberg, Peter Friedrich, Alexis Finoguenov, Günther Hasinger, Eckhard Kendziorra, Ingo Kreykenbohm, Christian Schmid, Jörn Wilms, Georg Lamer, Norbert Meidinger, Martin Mühlegger, Mikhail Pavlinsky, Jan Robrade, Andrea Santangelo, Jürgen Schmitt, Axel Schwope, Matthias Steinmetz, Lothar Strüder, Rashid Sunyaev, Chris Tenzer, “eROSITA on SRG,” May 2010, URL: http://arxiv.org/PS_cache/arxiv/pdf/1001/1001.2502v2.pdf
47) N. Meidinger, R. Andritschke, J. Elbs, O. Hälker, R. Hartmann, G. Hasinger, S. Herrmann, P. Holl, N. Kimmel, S. Müller,P. Predehl, G. Schächner, H. Soltau, L. Strüder, L. Tiedemann, “eROSITA camera design and first performance measurements with CCDs,” Proceedings of SPIE, 'Space Telescopes and Instrumentation 2008: Ultraviolet to Gamma Ray,' edited by Martin J. L. Turner, Kathryn A. Flanagan, Marseille, France, June 23-28, 2008, Vol. 7011, pp. 70110J-1 to 70110J-12
48) J. Treis, R. Andritschke, R. Hartmann, S. Herrmann, P. Holl, T. Lauf, P. Lechner, G. Lutz, N. Meidinger, M. Porro, R. H. Richter, F. Schopper, H. Soltau, L. Strüder, “Pixel detectors for x-ray imaging spectroscopy in space,” Pixel 2008 International Workshop, FermiLab, Batavia, IL, USA, Sept. 23-26, 2008, URL: http://www.iop.org/EJ/article/1748-0221/4/03/P03012/jinst9_03_p03012.pdf?request-id=54a4fd96-1512-4cdc-9452-e0791533bee8
49) http://www.mpe.mpg.de/erosita/
50) “eROSITA approved, April 1, 2007, URL: http://www.hll.mpg.de/02_news/PM07_eRosita/PM_01-07_erosita_approved.pdf
51) http://www.aip.de/People/ASchwope/papers/eROSITA_SPIE_2006.pdf
52) Peter Predehl on behalf of the eROSITA team, “eROSITA,” 2007, URL: http://universe-cluster.de/MaKaC/getFile.py/access?contribId=13&resId=0&materialId=slides&confId=245
53) P. Predehl, G Hasinger, H. Böhringer, U. Briel, H. Brunner, E. Churazov, M. Freyberg, P. Friedrich, E. Kendziorra, D. Lutz, N. Meidinger, M. Pavlinsky, E. Pfeffermann, A. Santangelo, J. Schmitt, P. Schuecker, A. Schwope, M. Steinmetz, L. Strüder, R. Sunyaev, J. Wilms, “eROSITA,” Proceedings of SPIE, Vol. 6266, pp. 62660P-1-62660P-10, URL: http://www.aip.de/People/ASchwope/papers/eROSITA_SPIE_2006.pdf
54) N. Cappelluti, P. Predehl, H. Böheringer, H. Brunner, M. Brusa, V. Burwitz, Evgeniy Churazov, K. Dennerl, M. Freyberg, P. Friedrich, G. Hasinger, E. Kenziorra, I. Kreykenbohm, G. Lamer, N. Meidinger, M. M¨uhlegger, M. Pavlinsky, J. Robrade, A. Santangelo, J. Schmitt, A. Schwope, M. Steinmitz, L. Strüder, R. Sunyaev, C. Tenzer, “eROSITA on SRG - A X-ray all-sky survey mission,” Journal of the Italian Astronomical Society, SAIt 2008, URL: http://arxiv4.library.cornell.edu/PS_cache/arxiv/pdf/1004/1004.5219v1.pdf
55) Peter Predehl, “eROSITA - An introduction to instrument and mission,” First eROSITA International Conference, Garmisch-Partenkirchen, Germany, Oct. 17-20, 2011, URL: http://www.mpe.mpg.de/erosita/erosita2011/program/PDF/predehl.pdf
56) http://www.mpe.mpg.de/erosita/erosita2011/program/default.html
57) A. Merloni, “The eROSITA Bulletin,” No 3, May 2013, MPE, URL: http://www.mpe.mpg.de/5098212/eROSITA_Bulletin3.pdf
58) Peter Friedrich on behalf of the eROSITA team, “eROSITA X-ray optics,” First eROSITA International Conference, Garmisch-Partenkirchen, Germany, Oct. 17-20, 2011, URL: http://www.mpe.mpg.de/erosita/erosita2011/program/PDF/friedrich.pdf
59) “Frame Store pnCCD for eROSITA,” URL: http://www.hll.mpg.de/graphics/PDF_BrochArticle/MPI-HLL-brochure_7_2.pdf
60) A. Merloni, “The eROSITA Bulletin,” No 4, February 2014, URL: http://www.mpe.mpg.de/5394374/eROSITA_Bulletin4.pdf
61) A. Merloni, “The eROSITA Bulletin,” No 1, July 2012, MPE, URL: http://www.mpe.mpg.de/5098186/eROSITA_Bulletin1.pdf
62) M. Pavlinsky, V. Akimov, V. Levin, I. Lapshov, A. Tkachenko, N. Semena, V. Arefiev, A. Glushenko, A. Yaskovich, R. Burenin, S. Sazonov, M. Revnivtsev, M. Buntov, S. Grebenev, A. Lutovinov, M. Kudelin, S. Grigorovich, D. Litvin, V. Lazarchuk, I. Roiz, M. Garin, M. Gubarev, B. Ramsey, K. Kilaru, S. L. O'Dell, R. Elsner , “The ART-XC Instrument on board the SRG Mission,” Proceedings of SPIE, Vol. 8147, 814706 (2011); doi:10.1117/12.895229 URL: http://ntrs.nasa.gov/archive/nasa/casi.ntrs.nasa.gov/20110015817_2011016706.pdf
63) ”Spectrum RG,” IKI, URL: http://www.iki.rssi.ru/eng/srg.htm
64) M. Gubarev, B. Ramsey, S. L. O’Dell, R. Elsner, K. Kilaru, J. McCracken, M. Pavlinsky, A. Tkachenko, I. Lapshov, “The Marshall Space Flight Center Development of Mirror Modules for the ART-XC Instrument aboard the Spectrum-Roentgen-Gamma Mission,” URL: http://ntrs.nasa.gov/archive/nasa/casi.ntrs.nasa.gov/20120015346.pdf
65) M. Gubarev, B. Ramsey, S. L. O’Dell, R. Elsner, K. Kilaru, J. McCracken, M. Pavlinsky, A. Tkachenko, I. Lapshov, C. Atkins, V. Zavlin, “Development of Mirror Modules for the ART-XC Instrument aboard the Spectrum-Roentgen-Gamma Mission,” Proceedings of SPIE, 'Optics for EUV, X-Ray, and Gamma-Ray Astronomy VI,' Vol. 8861, Sept. 26, 2013, URL: http://ntrs.nasa.gov/archive/nasa/casi.ntrs.nasa.gov/20140005793.pdf
66) B. Ramsey, M.Gubarev, R.Elsner, J.Kolodziejczak, S.O’Dell, D.Swartz, M. Pavlinsky, A. Tkachenko, I. Lapshov, “Development and Calibration of the ART-XC Mirror Modules for the Spectrum Rontgen Gamma Mission,” Sept. 2013, URL: http://ntrs.nasa.gov/archive/nasa/casi.ntrs.nasa.gov/20140006455.pdf
67) M. Gubarev, B. Ramsey, J. J. Kolodziejczak, S. L. O'Dell, R. Elsner, V. Zavlin, D. Swartz, M. Pavlinsky, A. Tkachenko, I. Lapshov, “The calibration of flight mirror modules for the ART-XC instrument on board the SRG mission,” Proceedings of SPIE, 'Space Telescopes and Instrumentation 2014: Ultraviolet to Gamma Ray', Vol. 9144, Montreal, Canada, July 22-27, 2014
68) Lee Mohon, ”Spectrum X-Gamma Rockets into Space with X-ray Vision,” NASA, 15 July 2019, URL: https://www.nasa.gov/centers/marshall/news/news/releases/2019/spectrum-x-gamma-rockets-into-space-with-x-ray-vision.html
The information compiled and edited in this article was provided by Herbert J. Kramer from his documentation of: ”Observation of the Earth and Its Environment: Survey of Missions and Sensors” (Springer Verlag) as well as many other sources after the publication of the 4th edition in 2002. - Comments and corrections to this article are always welcome for further updates (eoportal@symbios.space).
Spacecraft Launch Mission Status Sensor Complement References Back to top