OTB-1 (Orbital Test Bed-1)
EO
Operational (nominal)
SSTL
Quick facts
Overview
Mission type | EO |
Agency | SSTL |
Mission status | Operational (nominal) |
Launch date | 25 Jun 2019 |
CEOS EO Handbook | See OTB-1 (Orbital Test Bed-1) summary |
OTB-1 (Orbital Test Bed-1)
Spacecraft Launch Mission Status Sensor Complement References
Overview
After working with a wide range of potential users, Surrey has finalized the OTB-1 flight manifest. The OTB-1 satellite will carry five demonstration payloads for a variety of commercial, government, and academic organizations. With the assistance of the Department of Defense STP (Space Test Program), OTB is scheduled to launch on the SpaceX Falcon Heavy. The OTB-1 satellite is the first true commercial all hosting satellite and the first spacecraft to be integrated at SST-US (Surrey Satellite Technology-US LLC), the new Surrey US facility in Englewood, Colorado. 1)
Hosted payload missions play a critical role in the development of new aerospace technologies. The OTB-1 satellite provides aerospace organizations with a low-risk opportunity to test new subsystems and payload technologies on an actual low-Earth orbit mission while sharing the cost of development and launch. The rideshare concept behind OTB-1 is a cost-effective way to rapidly space-qualify new equipment and generate in-orbit data.
The primary hosted payload of OTB-1 is DSAC (Deep Space Atomic Clock) of NASA/JPL (Jet Propulsion Laboratory), Pasadena, CA. DSAC is a miniaturized, ultra-precise mercury-ion atomic clock that is orders of magnitude more stable than current spaceborne navigation clocks. 2)
• Already in 2012, JPL inquired about a hosting position for their DSAC instrument. DSAC had recently lost its hosted position on IRIDIUM and was increasingly looking for hosting opportunities as the payload was maturing and ready for a flight demonstration needed to increase its TRL (Technology Readiness Level) from 5 to 7 (Ref. 7).
• In July 2013, SST-US announced that NASA/JPL had selected SST-US for the flight of the Deep Space Atomic Clock (DSAC) payload under the sponsorship of NASA's STMD (Space Technology Mission Directorate). DSAC will fly on the SST-US-owned-and-operated OTB (Orbital Test Bed) satellite. 3)
- Under the agreement SST-US will provide a hosted payload flight opportunity for the NASA DSAC payload on its upcoming OTB mission, scheduled for launch during 2015. DSAC features a miniaturized, ultra-precise mercury-ion atomic clock which is an order of magnitude more stable than today’s best space-based navigation clocks. In-orbit demonstration of the precision timing and navigation capabilities of the DSAC instrument is a key requirement for NASA’s pursuit of deep space exploration missions which require higher-precision data collection and autonomous radio navigation for time-critical events such as orbit insertion or landing.
• In July 2014, the SST-US has been awarded a firm-fixed-price IDIQ (Indefinite-Delivery/Indefinite-Quantity) contract under the HoPS (Hosted Payload Solutions) program from the U.S. Air Force SMC (Space and Missile Systems Center) Contracting Directorate. 4)
- SMC qualified SST-US as a vendor to meet the government's needs for various hosted payload missions. The HoPS IDIQ contract will provide a rapid and flexible means for the government to acquire commercial hosting capabilities for government payloads.
- Within the HoPS IDIQ scope, SST-US will provide hosted payload studies and hosted payload missions. The HoPS studies include those study activities related to enabling hosted payloads. The missions will encompass fully-functioning on-orbit hosted payload space and ground systems for government-furnished payloads on commercial platforms. In addition to the space and ground systems, the HoPS missions will include related on-orbit support for data transfer from the hosted payload to the government end user(s).
Spacecraft
Owned and operated by Surrey US, the OTB-1 satellite is based on the CFESat (Cibola Flight Experiment Satellite) modular design developed by the Surrey group for LANL (Los Alamos National Laboratory) using the flight-proven SSTL-150 satellite bus. The Surrey engineering team in Colorado will utilize the same satellite integration methodologies developed by the Surrey group over a period of three decades that have resulted in 41 successful operational and experimental satellite missions. The nominal OTB spacecraft configuration and on-orbit orientation are shown in Figure 1. 5) 6) 7)
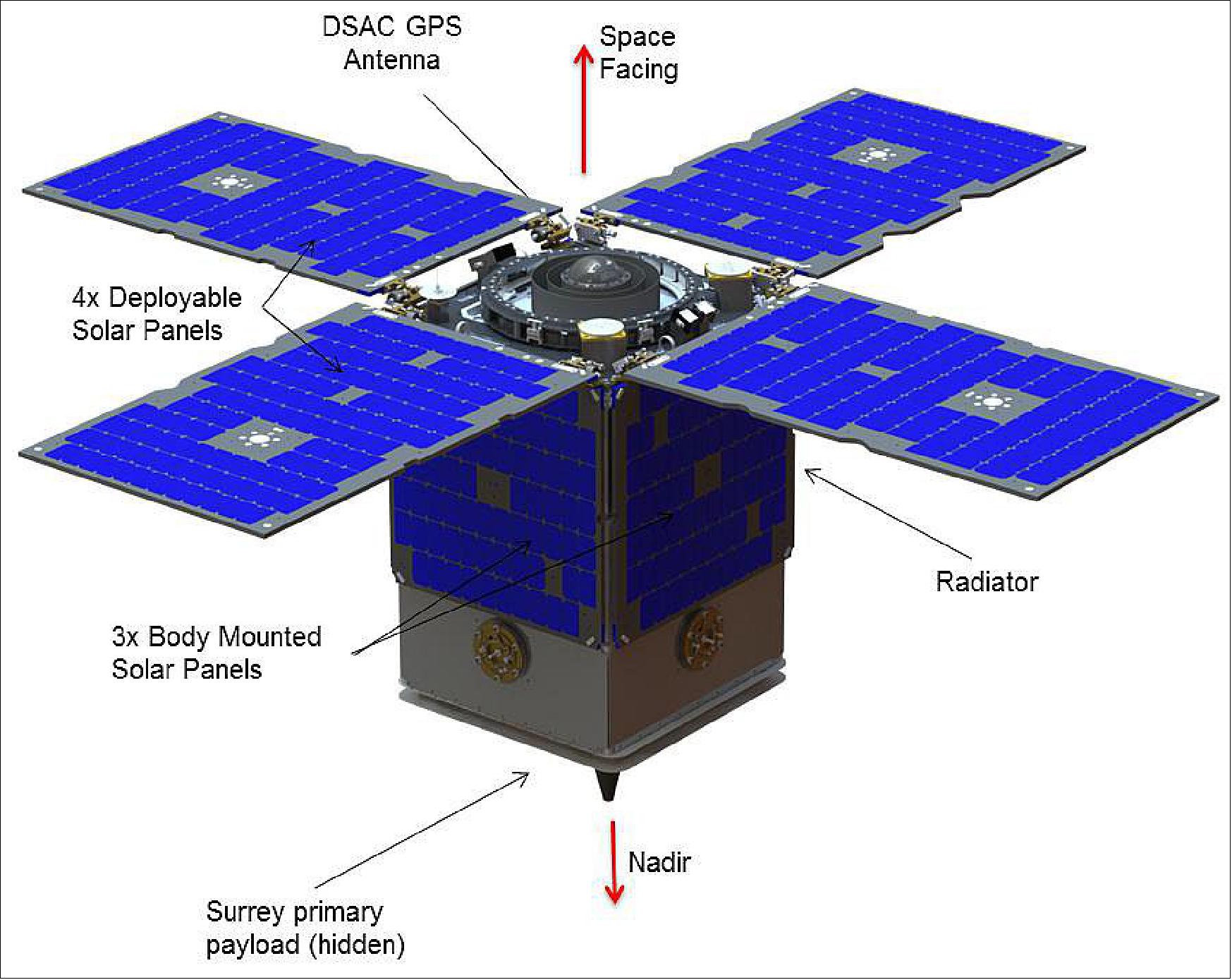

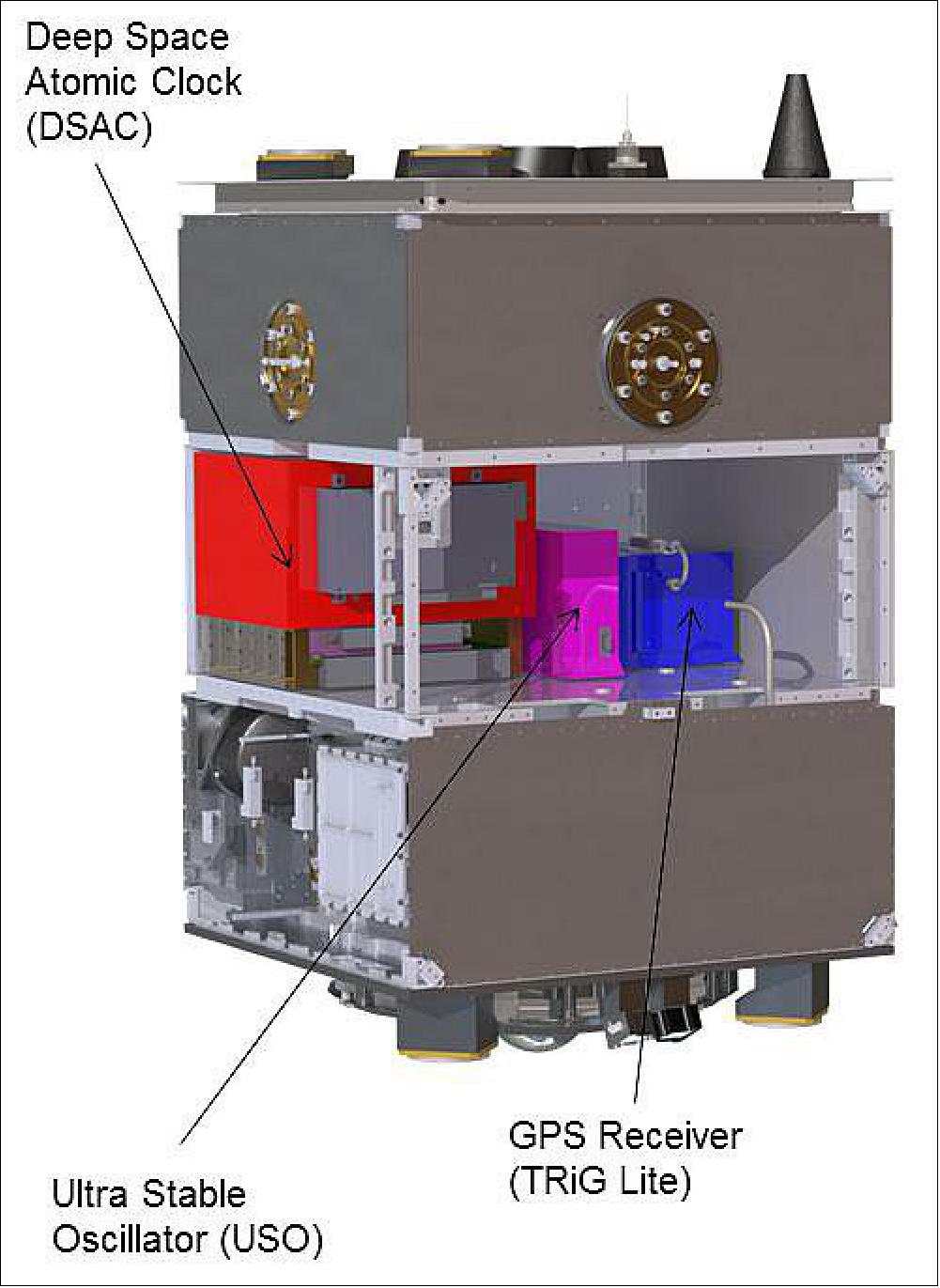
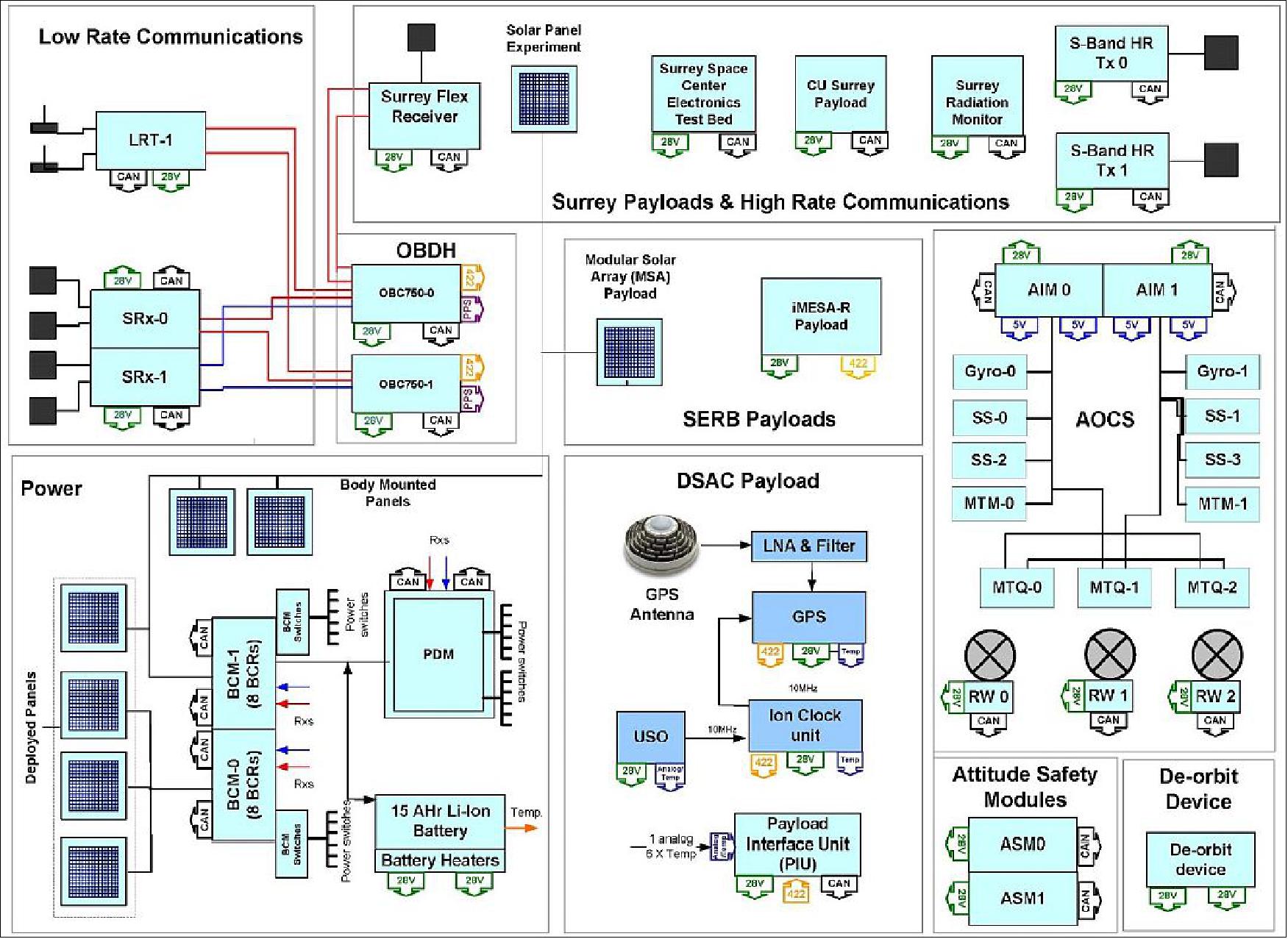
The OTB spacecraft has been designed for nadir pointing. The DSAC space-pointing requirements for the GPS antenna to be zenith-oriented represent a nice complement to that primary attitude (Figure NO TAG#). Some other key characteristics of OTB as they relate to DSAC on-orbit investigation follow:
Attitude knowledge and control: The spacecraft provides a relatively stable platform for the DSAC investigation. The spacecraft has no active propulsion system. All deployables (such as the solar arrays) are fixed once deployed. Deorbit at the end of the OTB mission is accomplished by a sail. The ADCS (Attitude Determination and Control Subsystem ) utilizes wheels, gyroscopes, and a magnetic control system utilizing magnetorquers and a magnetometer. Magnetic effects on the clock, from the magnetorquers and the Earth’s magnetic field, have been modeled and are not expected to be a significant error source for DSAC’s long-term stability. Twice during the one-year mission, the spacecraft rotates by 180º about the Z-axis (yaw flip) to maximize the solar array exposure and to minimize the exposure of the spacecraft radiator to the sun in response to seasonal variations in the environment. The clock is expected to operate through these maneuvers. Attitude knowledge is achieved via the magnetometer, gyroscopes, and the four sun sensors. The DSAC team receives the vehicle’s attitude history via the same file-based transfer interface used for the DSAC payload and GPS telemetry. This data is available within approximately twenty-four hours of the payload and GPS telemetry.
TCS (Thermal Control Subsystem): While the spacecraft will experience significant temperature variations throughout the year-long mission and more modest variations during any given orbit, the thermal interfaces to the USO Ultra Stable Oscillator, clock, and GPS receiver have been designed so that the expected thermal variations meet their thermal operational range (with potentially short outages during the peak heating season). There are no specific requirements on thermal control of the cabling between the units or the GPS antenna. A particular thermal sensitivity relevant to the validation system is the GPS receiver’s phase sensitivity to temperature variations.
Power: Spacecraft power is provided by the solar panels. The orientation of the solar panels has been selected to minimize multi-path effects on the GPS antenna, and their configuration is considered in the spacecraft model used by the precision clock determination process. The DSAC payload is required to operate across a wide input voltage range, but DSAC power converters, that manage the voltage beyond the interface, regulate the applied voltage sufficiently that changes in the input voltage are not expected to be a factor in PCD.
Incorporated into the satellite power system are solar cells designed by EMCORE Corp. of Albuquerque, NM, that are more efficient than those flown on previous Surrey missions.
Time: The OTB clock (not DSAC) is used to time-tag all platform telemetry (such as the attitude history). The OTB ground system will synchronize the on-board clock with a ground-based reference nominally once per day, and the ground is synched to UTC using a GPS receiver and a time server. The on-board computer sends that time out periodically to the various spacecraft nodes and other payload units that require it. The inaccuracies in these time tags relative to GPS time are small and introduce second order effects in the DSAC data analysis. (Note that the GPS receiver data is time-tagged using the GPS receiver clock). Any discontinuities in the telemetry time-tags with GPS time will be resolved by the DSAC ground system.
Figure 5 illustrates the on-orbit mission architecture, where the plan is to collect measurements between GPS and the host spacecraft using a GPS receiver connected to DSAC. The data will be processed on the ground by the DSAC team to validate the clock’s in-space performance.
A key objective of the project is to demonstrate DSAC’s performance and operability in space, and develop the technology to a point where it can be transitioned to industry for commercial production. DSAC’s space demonstration will have proven its flight worthiness and will radically reduce risk to future missions that adopt it.
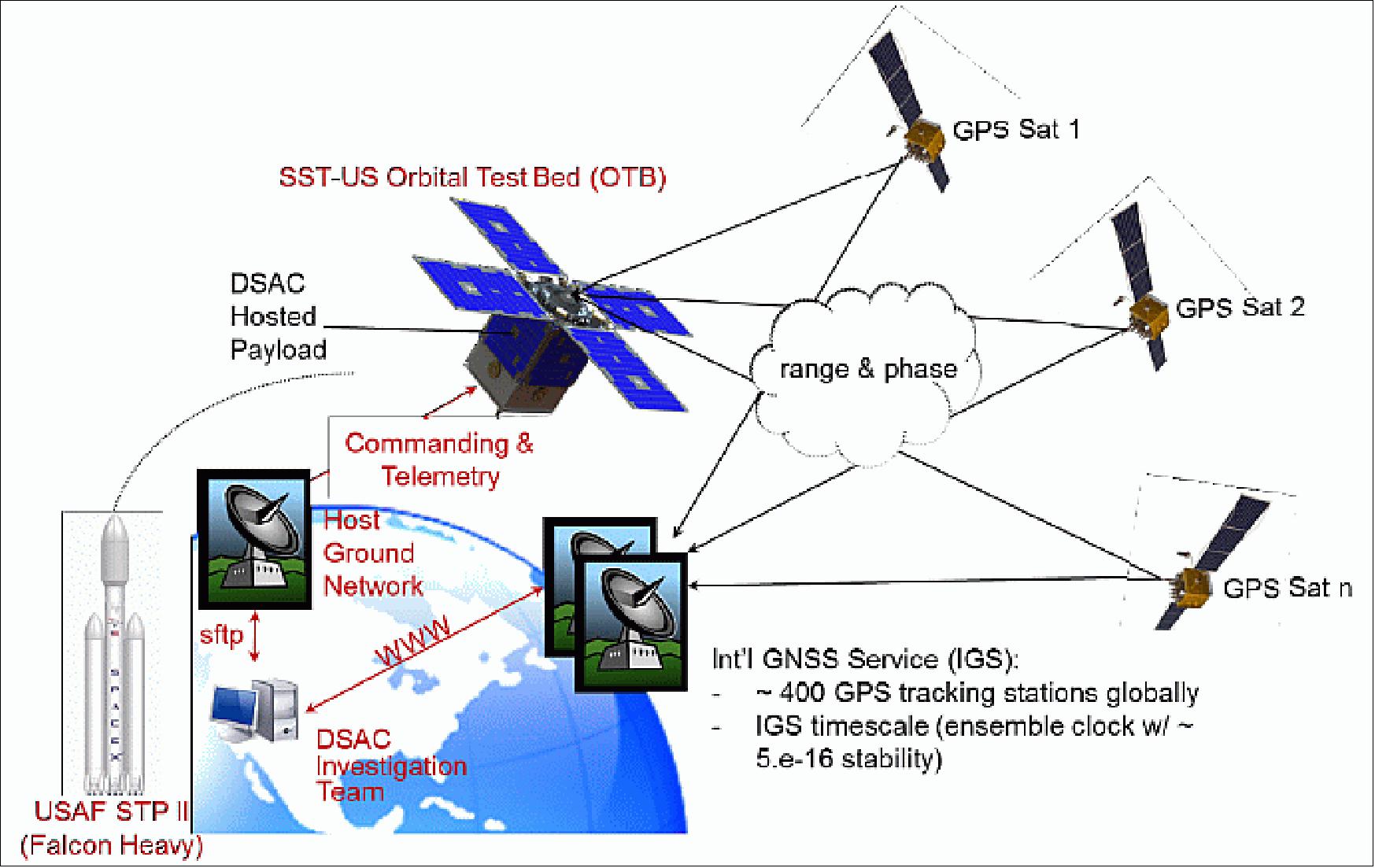
GN&C (Guidance Navigation and Control) changes and more:
The rideshare launch opportunity carried with it a specified and perhaps disadvantageous orbit of 720 km and 24º inclination. This put constraints on the TT&C (Tracking Telemetry and Control) and power as most missions, this bus was originally designed for are sun synchronous and most Surrey ground stations are at high latitudes. A yaw maneuver will also be required every 6 months in this orbit. Propulsion has been removed as orbit maintenance will not be required.
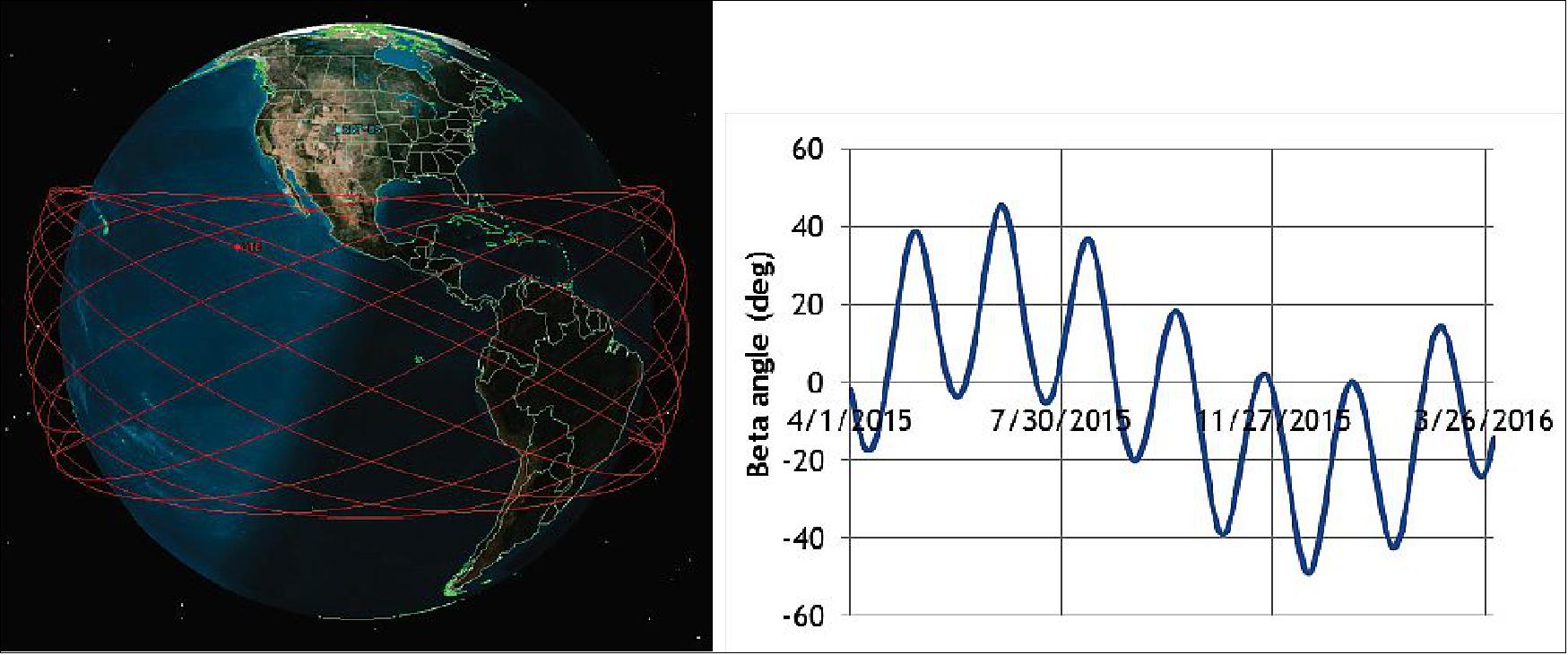
For the lower inclination, the limitation of a 5 minute TT&C pass per day, and the accommodations needed for the payloads has driven additional changes to the GN&C. There has been power negative spins identified that have to be avoided upon launch tip off and possible loss of control and communications through life. The following are some of the avionics changed or added:
• STIM-210 gyros (Sensonor Technologies) for pointing during eclipse
• Two ASMs (Active Safety Monitors)
• Moog Bradford sun sensors
The Active Safety Monitors are used in the case of an anomaly and will control OTB-1. The watch dog timer going off will turn these units on with redundant unit cold. Only the ASMs, AIM, X and Y wheels and heaters will be powered to regain safe mode. Given the removal of the standard star trackers and the above modifications, OTB-1 has the control specifications as shown in Table 1.
Sunlit case | Roll (º,1σ) | Pitch (º,1σ) | Yaw (º,1σ) |
Knowledge error - requirement | 0.600 | 0.800 | 0.600 |
Eclipse case | Roll (º, mean + 1σ) | Pitch (º, mean + 1σ) | Yaw (º, mean + 1σ) |
Knowledge error - requirement | 1.500 | 1.500 | 1.500 |
GPS (Global Positioning System) receiver: The needs of DSAC required upgrading the typical SGR-10 GPS receiver with the TRIG-POD, which became part of the DSAC payload. Also, the GPS antenna with choke rings (to minimize multipath) has been mounted to the anti-nadir deck to give a maximum number of GPS satellites in view.
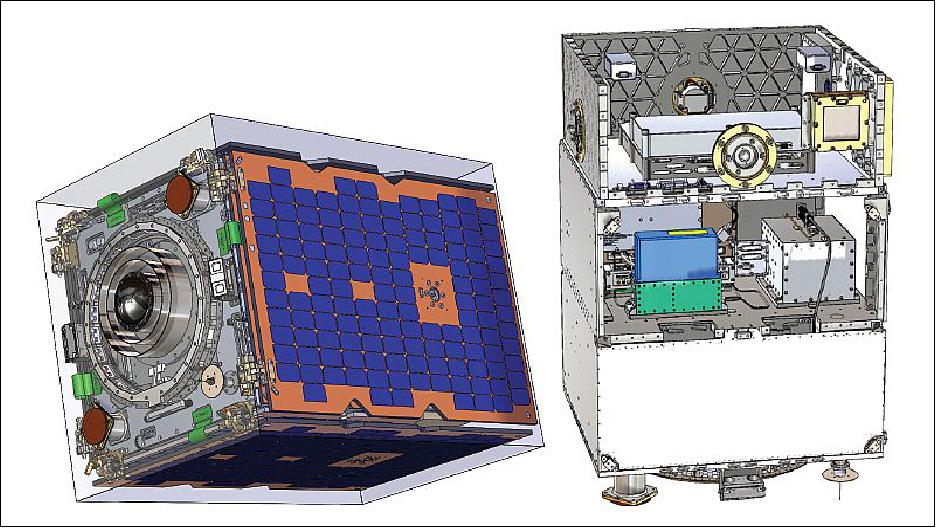
Magnetorquers: DSAC also needed a stable magnetic field environment and is shielded for this purpose, but the magnetorquer induced fields still required studied to make sure they were below the sensitivity levels of the payload. With the flexibility of the layout the magnetorquers were moved away from the atomic clock and an analysis was done to show that the simulated fields were tolerable.
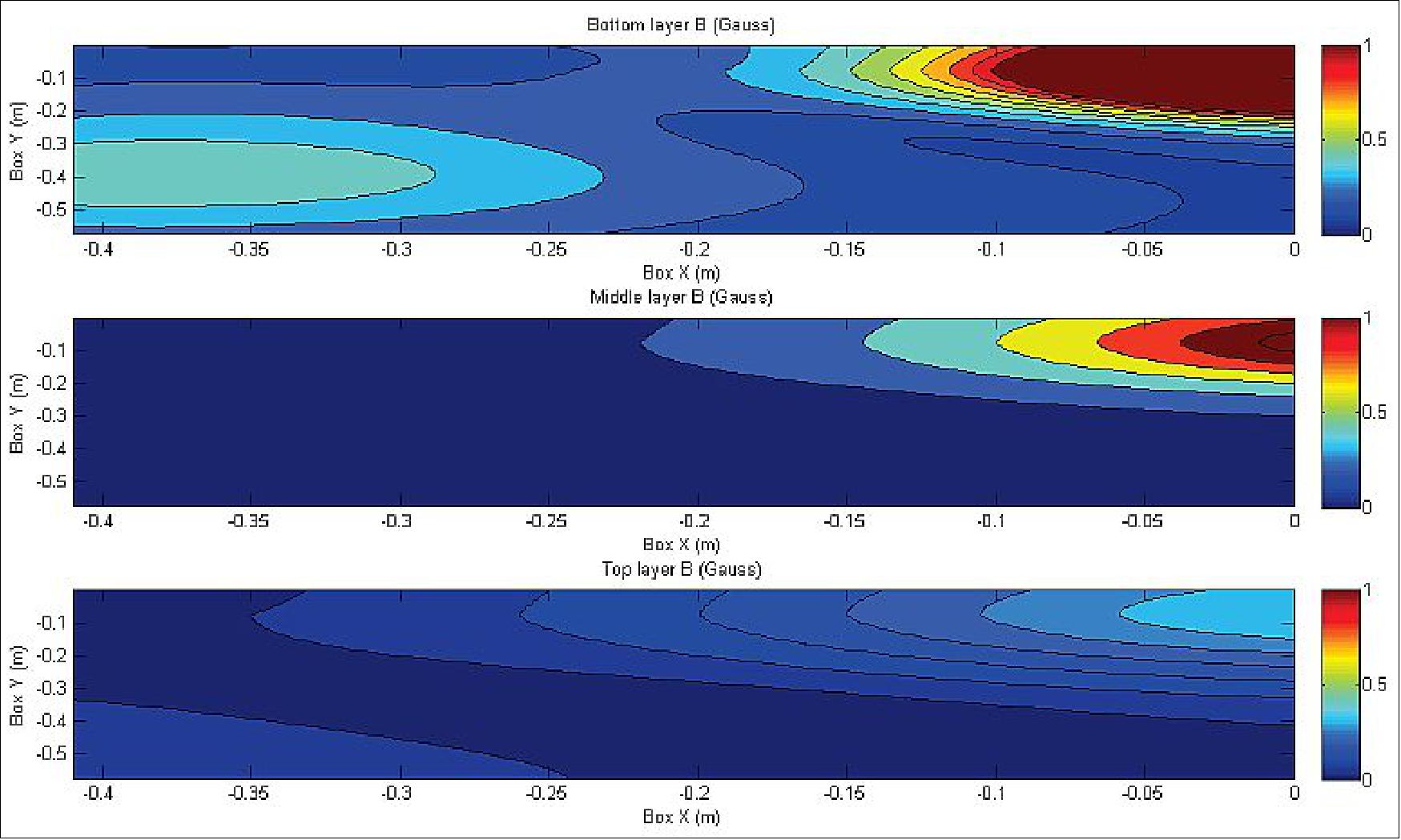
Other changes: The reaction wheels were taken from 4 to 3. Maneuvers and safe modes can be accomplished with 2 wheels and torque rods. The wheels also have a great on orbit heritage and this saves space. Propulsion was eliminated because of the relatively high orbit and no need to maintain it. On the other hand a deorbit device will be employed to make sure the bus will come down in the required time frame (25 years). The relatively small demand for downlink allowed Surrey to drop the X- band system and use a S-band system including the new High Rate system being tested on-board.
Development Status
• September 17, 2018: General Atomics Electromagnetic Systems (GA-EMS) announced today that it has been awarded a contract from NASA to host the agency’s Multi-Angle Imager for Aerosols (MAIA) instrument on a satellite designed by GA-EMS. NASA’s MAIA payload will be delivered and integrated on the Orbital Test Bed (OTB)-2 spacecraft at GA-EMS’ Englewood, CO facility. GA-EMS will perform services to include payload integration, satellite testing and MAIA pre-launch processing, spacecraft and launch vehicle coordination, in-orbit checkout, and on-orbit spacecraft operations enabling instrument operations. 8)
- “We’ve developed a very successful working relationship with the NASA team, hosting their Deep Space Atomic Clock (DSAC) on our Orbital Test Bed satellite,” stated Scott Forney, president of GA-EMS. “We are excited to be working with NASA’s team once again to apply our innovations in modular satellite design to support MAIA’s planned multi-year mission to gather critical data for researchers to analyze pollution’s effect on the human population.”
- GA-EMS’ hosted satellite services provide a unique opportunity for customers to launch experimental hardware and payloads into Earth orbit without incurring the overhead of commissioning a full satellite mission. GA-EMS provides a breadth of experience in small satellite mission experience and services to include early concepts through to on-orbit operations.
- “Our designs provide lower-cost access to space while helping customers keep pace with the demand to launch technologies like the MAIA and DSAC, to advance NASA’S research goals,” stated Nick Bucci, vice president for GA-EMS Missile Defense and Space Systems. “Our paradigm-shifting approach to small satellite design, coupled with our operational flight experience and focus on high mission assurance, allows us to create tailored solutions in a shorter timeframe.”
- Scheduled for launch in 2022, MAIA’s planned three to five year on-orbit operation will measure particulate matter to allow correlation with adverse human health issues such as cardiovascular and respiratory disease. MAIA is a Venture-class investigation within NASA’s Earth System Science Pathfinder Program at the agency’s Langley Research Center in Virginia. NASA’s Jet Propulsion Laboratory in Pasadena, CA is responsible for MAIA instrument design, development, and delivery.
• April 23, 2018: General Atomics Electromagnetic Systems (GA-EMS) announced today that it has completed full system and “ready for launch” pre-flight testing of its Orbital Test Bed (OTB) satellite. OTB will launch as part of the U.S. Air Force’s Space Technology Program (STP-2) flight on the SpaceX Falcon Heavy rocket. The OTB hosts multiple payloads on a single platform for on-orbit technology demonstration. Among the hosted payloads on OTB is NASA’s Space Technology Mission Directorate’s DSAC (Deep Space Atomic Clock), designed and built at NASA’s Jet Propulsion Laboratory, which supports deep space navigation and exploration. 9)
- “The completion of system testing marks a significant milestone, allowing us to effectively “button up” the OTB satellite in anticipation of delivery to Cape Canaveral for launch into space,” stated Scott Forney, president of GA-EMS. “We believe OTB is a new paradigm in hosted payload satellite design and is paving the way to make space more affordable and accessible to customers looking to demonstrate and validate their technologies on-orbit.”
• In November 2017, General Atomics of San Diego announced that it had acquired the majority of the assets of Surrey Satellite Technology US LLC (Surrey-US), an Englewood, Colorado-based provider of innovative small satellite technologies, systems and services. The assets and workforce will be integrated into General Atomics' Electromagnetic Systems Group (GA-EMS) to support the organization's growth initiatives focused on the development and delivery of small satellite and advanced payload systems. 10)
- "We look forward to the Surrey-US team joining us as we continue to expand our portfolio of small satellite and mission-support capabilities," stated Scott Forney, president of GA-EMS. "The Surrey-US team's expertise in designing and deploying small satellites and end-to-end mission solutions is complementary to ours. We are excited that GA-EMS now will offer ground-to-space solutions utilizing nano, micro and small satellites from 3U to 500 kg with a high degree of modularity and payload flexibility.
- Surrey-US was established in 2008 as a wholly-owned subsidiary of UK-based Surrey Satellite Technology Ltd. (SSTL) to address the U.S. market and its core capabilities include the complete design, manufacture, launch and operation of small satellites. SSTL is a world-renowned leader in the design, development and deployment of small satellites, with a 35 year legacy of successful launches, and a proven track record in pioneering and delivery of small satellite and complete mission solutions for defense, telecommunications, earth observation, and technology demonstrations.
- GA-EMS is a global leader in the research, design, and manufacture of first-of-a-kind electromagnetic and electric power generation systems. GA-EMS' research, development and technology innovation has led to an expanding portfolio of specialized products and integrated system solutions supporting aviation, space systems and satellites, missile defense, power and energy, and processing and monitoring applications for critical defense, industrial, and commercial customers worldwide.
Launch
OTB-1 is a secondary payload on the STP-2 rideshare mission of USAF, launched on 25 June 2019 (06:30 UTC) aboard a SpaceX Falcon Heavy launch vehicle from Launch Complex 39A at NASA’s Kennedy Space Center. The STP-2 payload includes six FormoSat-7/COSMIC-2 satellites (primary payload), developed by NOAA and Taiwan’s National Space Organization to collect GPS radio occultation data for weather forecasting. The mission also carries several NASA technology demonstrations. The STP-2 mission is led by the Air Force Space Command’s Space and Missile Systems Center (SMC). The total IPS (Integrated Payload Stack) has a mass of 3700 kg. 11)
The secondary payloads on this flight are:
• DSX (Demonstration and Science Experiments) mission of AFRL
• GPIM (Green Propellant Infusion Mission), a demonstration minisatellite of NASA (~180 kg). 12)
• FalconSat-7, a 3U CubeSat mission developed by the Cadets of the U.S. Air Force Academy (USAFA) at Colorado Springs, CO.
• NPSat-1 (Naval Postgraduate School Satellite-1) of the Naval Postgraduate School, Monterey, CA. A microsatellite of 86 kg.
• OCULUS-ASR (OCULUS-Attitude and Shape Recognition), a microsatellite (70 kg) of MTU (Michigan Technological University), Houghton, MI, USA.
• Prox-1, a microsatellite (71 kg) of SSDL (Space Systems Design Laboratory) at Georgia Tech.
• LightSail-2 of the Planetary Society, a nanosatellite (3U CubeSat, 5 kg) will be deployed from the parent satellite Prox-1.
• ARMADILLO of UTA (University of Texas at Austin), a nanosatellite (3U CubeSat) of ~ 4 kg.
• E-TBEx (Enhanced Tandem Beacon Experiment), a tandem pair (3U CubeSats) of SRI International.
• TEPCE (Tether Electrodynamics Propulsion CubeSat Experiment), a 3U CubeSat (3 kg) of NPS (Naval Postgraduate School).
• CP-9 , a joint CP-9/StangSat experiment, which is a collaboration between PolySat at Cal Poly and the Merritt Island High School, and is sponsored by the NASA LSP (Launch Services Program). CP-9 is a 2U CubeSat while StangSat is a 1U CubeSat.
• PSat-2 (ParkinsonSAT), a student built 1.5U CubeSat of USNA (US Naval Academy) with a mass of 2 kg.
• BRICSAT-2, a student built 1.5U CubeSat of USNA (US Naval Academy) to demonstrate a µCAT electric propulsion system and carry a ham radio payload.
• OTB-1 (Orbital Test Bed-1) a minisatellite developed by SSTL (based on the SSTL-150 bus, 138 kg) and owned by GA-EMS (General Atomics Electromagnetic Systems) of San Diego. One of the hosted payloads is NASA's DSAC (Deep Space Atomic Clock), a technology demonstration mission with the goal to validate a miniaturized, ultra-precise mercury-ion atomic clock that is 100 times more stable than today’s best navigation clocks. 13)
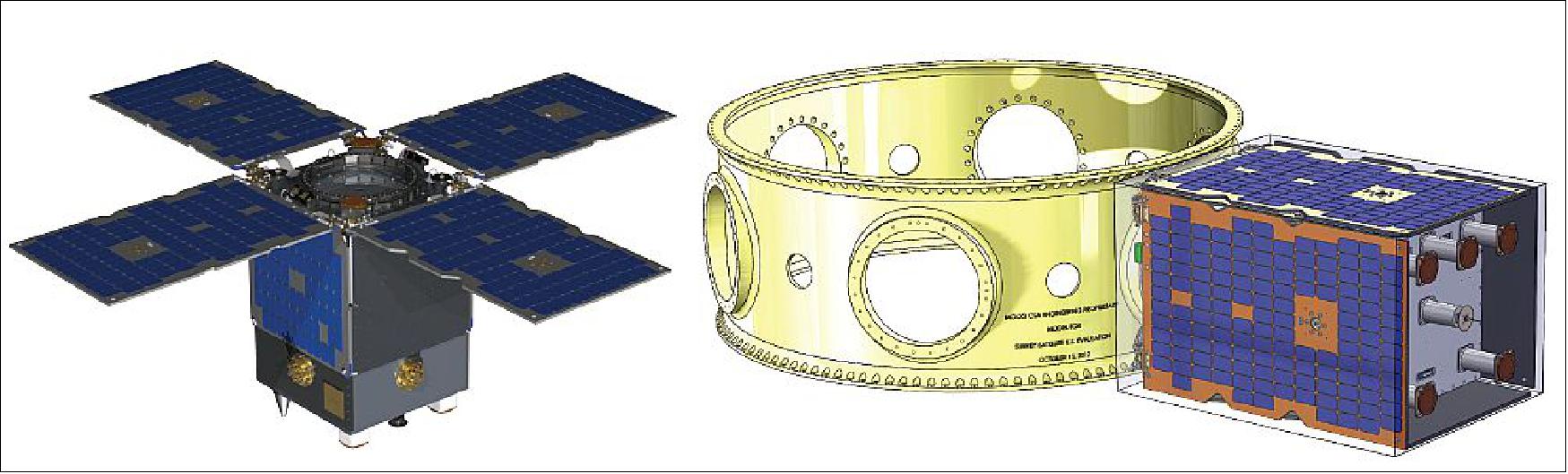
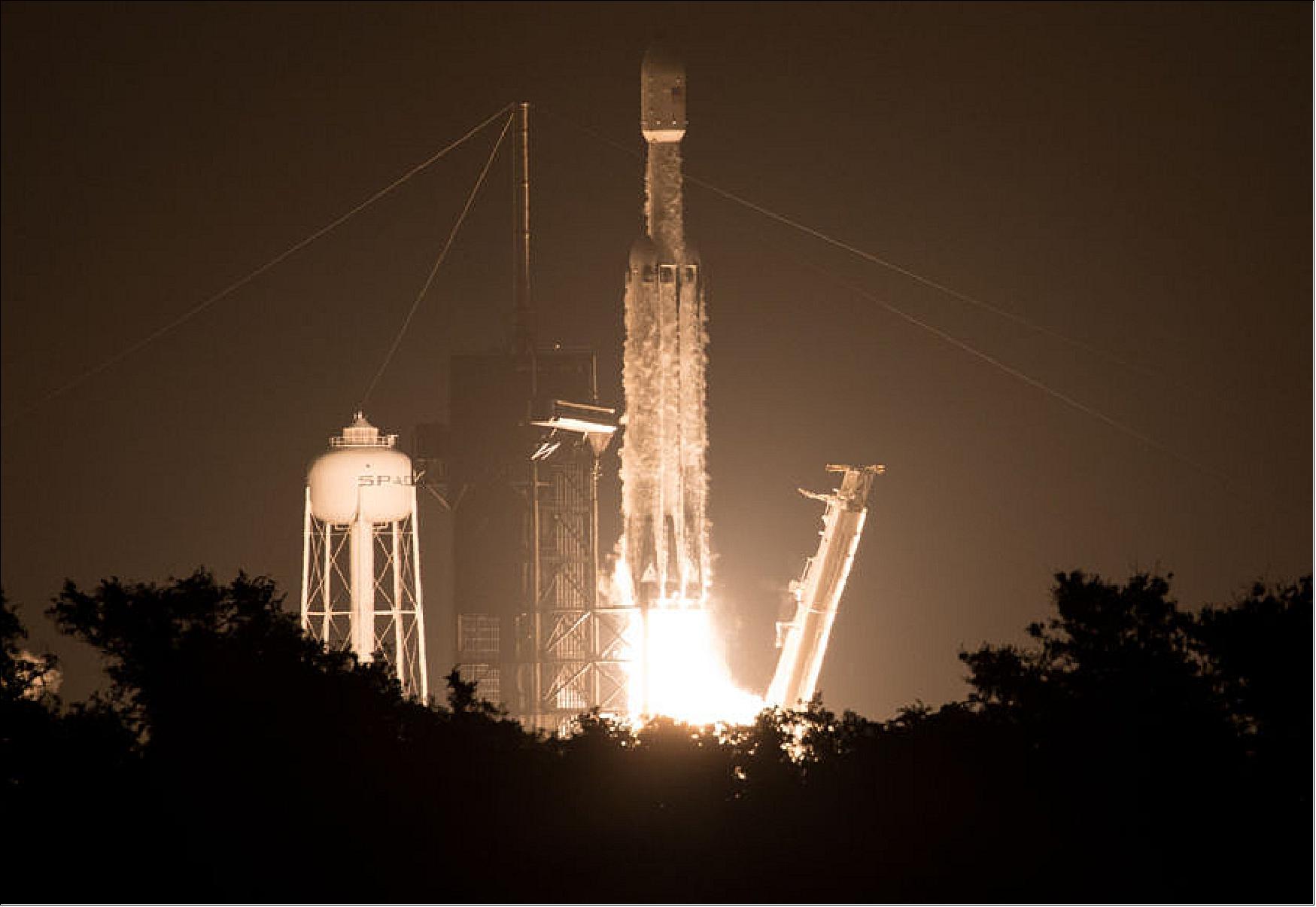
Orbits:
The STP-2 mission will be among the most challenging launches in SpaceX history with four separate upper-stage engine burns, three separate deployment orbits, a final propulsive passivation maneuver and a total mission duration of over six hours. It will demonstrate the capabilities of the Falcon Heavy launch vehicle and provide critical data supporting certification for future National Security Space Launch (NSSL) missions. In addition, [the USAF] will use this mission as a pathfinder for the [military’s systematic utilization of flight-proven] launch vehicle boosters.
The three orbits of the STP-2 mission for spacecraft deployment are:
1) The small secondary CubeSat satellites will be deployed into an elliptical orbit of ~300 x 860 km, inclination of ~28º. These are: OCULUS-ASR, TEPCE, E-TBEx, FalconSat-7, ARMADILLO, PSAT-2, BRICSAT, and CP-9/StangSat.
2) The second deployment batch of the STP-2 mission will occur at a circular altitude of 720 km and an inclination of 24º.
- Deployment of LightSail-2, Prox-1, and NPSat-1
- Deployment of OTB-1 with NASA's DSAC and GPIM
- The six FormoSat-7/COSMIC-2 satellites will be deployed into the initial circular parking orbit of 720 km. Eventually, they will be positioned in a low inclination orbit at a nominal altitude of ~520-550 km with an inclination of 24º (using their propulsion system). Through constellation deployment, they will be placed into 6 orbital planes with 60º separation.
3) The third and final deployment will be the Air Force Research Lab's DSX spacecraft as well as the ballast, which will be delivered to an elliptical MEO (Medium Earth Orbit) with a perigee of 6000 km and an apogee of 12000 km, inclination of 43º.
Mission Status
• October 5, 2021: For more than two years, NASA’s Deep Space Atomic Clock has been pushing the timekeeping frontiers in space. On Sept. 18, 2021, its mission came to a successful end. 15)
- The instrument is hosted on General Atomics’ Orbital Test Bed spacecraft that was launched aboard the Department of Defense Space Test Program 2 mission June 25, 2019. Its goal: to test the feasibility of using an onboard atomic clock to improve spacecraft navigation in deep space.
- Currently, spacecraft rely on ground-based atomic clocks. To measure a spacecraft’s trajectory as it travels beyond the Moon, navigators use these timekeepers to precisely track when those signals are sent and received. Because navigators know that radio signals travel at the speed of light (about 186,000 miles per second, or 300,000 km/s), they can use these time measurements to calculate the spacecraft’s exact distance, speed, and direction of travel.
- But the farther a spacecraft is from Earth, the longer it takes to send and receive signals – from several minutes to a few hours – significantly delaying these calculations. With an onboard atomic clock paired with a navigation system, the spacecraft could immediately calculate where it is and where it is going.
- Built by NASA’s Jet Propulsion Laboratory in Southern California, the Deep Space Atomic Clock is an ultra-precise, mercury-ion atomic clock encased in a small box that measures about 10 inches (25 cm) on each side – roughly the size of a toaster. Designed to survive the rigors of launch and the cold, high-radiation environment of space without its timekeeping performance degrading, the Deep Space Atomic Clock was a technology demonstration intended to carry out technological firsts and fill critical knowledge gaps.
- After the instrument completed its one-year primary mission in Earth orbit, NASA extended the mission to collect more data because of its exceptional timekeeping stability. But before the tech demo was powered off on Sept. 18, the mission worked overtime to extract as much data as possible in its final days.
- “The Deep Space Atomic Clock mission was a resounding success, and the gem of the story here is that the technology demonstration operated well past its intended operational period,” said Todd Ely, principal investigator and project manager at JPL.
- The data from the trailblazing instrument will help develop Deep Space Atomic Clock-2, a tech demo that will travel to Venus aboard NASA’s Venus Emissivity, Radio Science, InSAR, Topography & Spectroscopy (VERITAS) spacecraft when it launches by 2028. This will be the first test for an atomic clock in deep space and a monumental advancement for increased spacecraft autonomy.
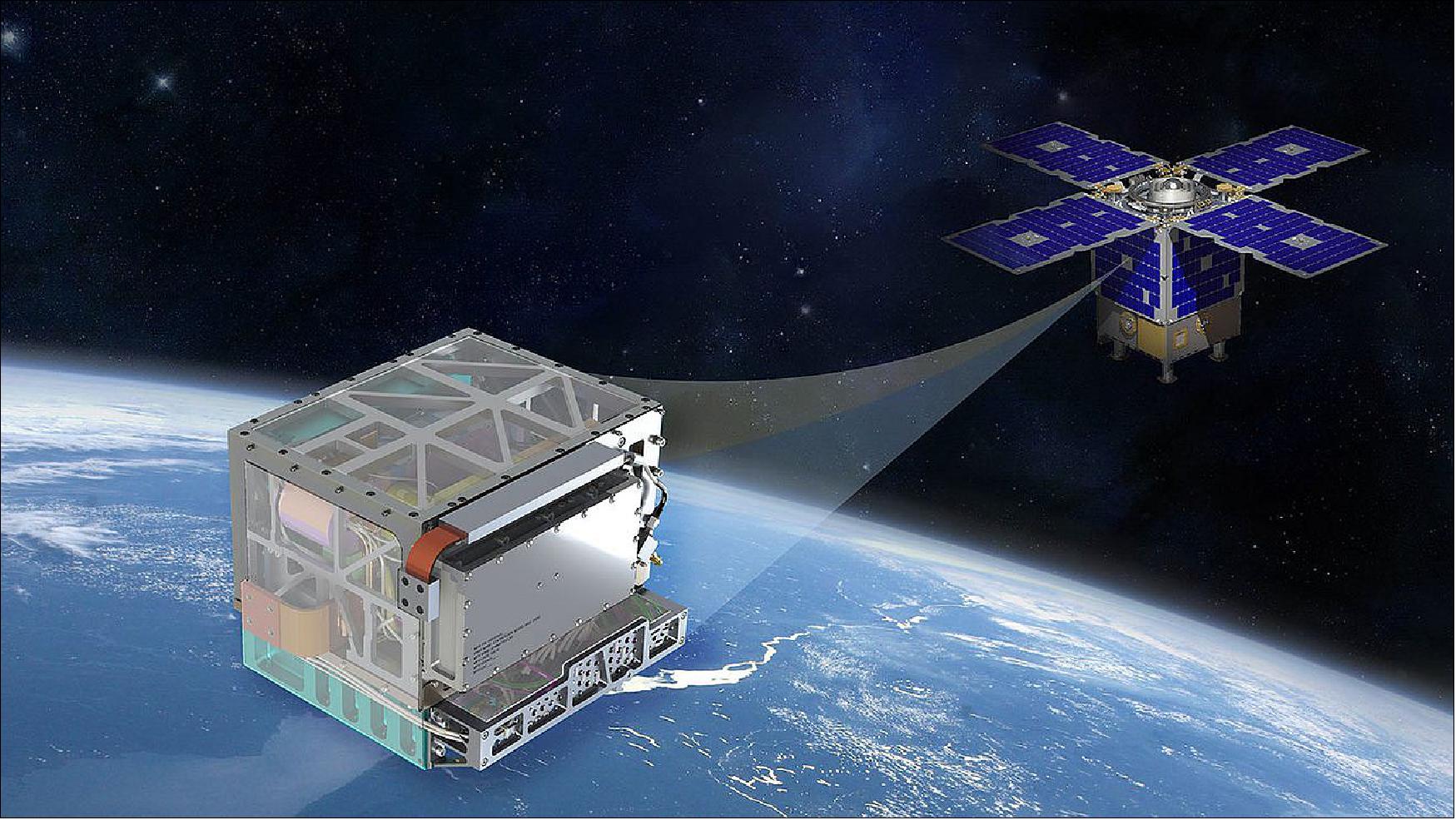
Stability Is Everything
- While atomic clocks are the most stable timekeepers on the planet, they still have instabilities that can cause a minuscule lag, or “offset,” in the clocks’ time versus the actual time. Left uncorrected, these offsets will add up and could lead to large errors in positioning. Fractions of a second could mean the difference between safely arriving at Mars or missing the planet altogether.
- Updates can be beamed from Earth to the spacecraft to correct for these offsets. Global Positioning System (GPS) satellites, for example, carry atomic clocks to help us get from point A to B. To make sure they keep the time accurately, updates need to be frequently transmitted to them from the ground. But having to send frequent updates from Earth to an atomic clock in deep space would not be practical and would defeat the purpose of equipping a spacecraft with one.
- This is why an atomic clock on a spacecraft exploring deep space would need to be as stable as possible from the get-go, allowing it to be less dependent on Earth to be updated.
- “The Deep Space Atomic Clock succeeded in this goal,” said JPL’s Eric Burt, an atomic clock physicist for the mission. “We have achieved a new record for long-term atomic clock stability in space – more than an order of magnitude better than GPS atomic clocks. This means that we now have the stability to allow for more autonomy in deep space missions and potentially make GPS satellites less dependent on twice-daily updates if they carried our instrument.”
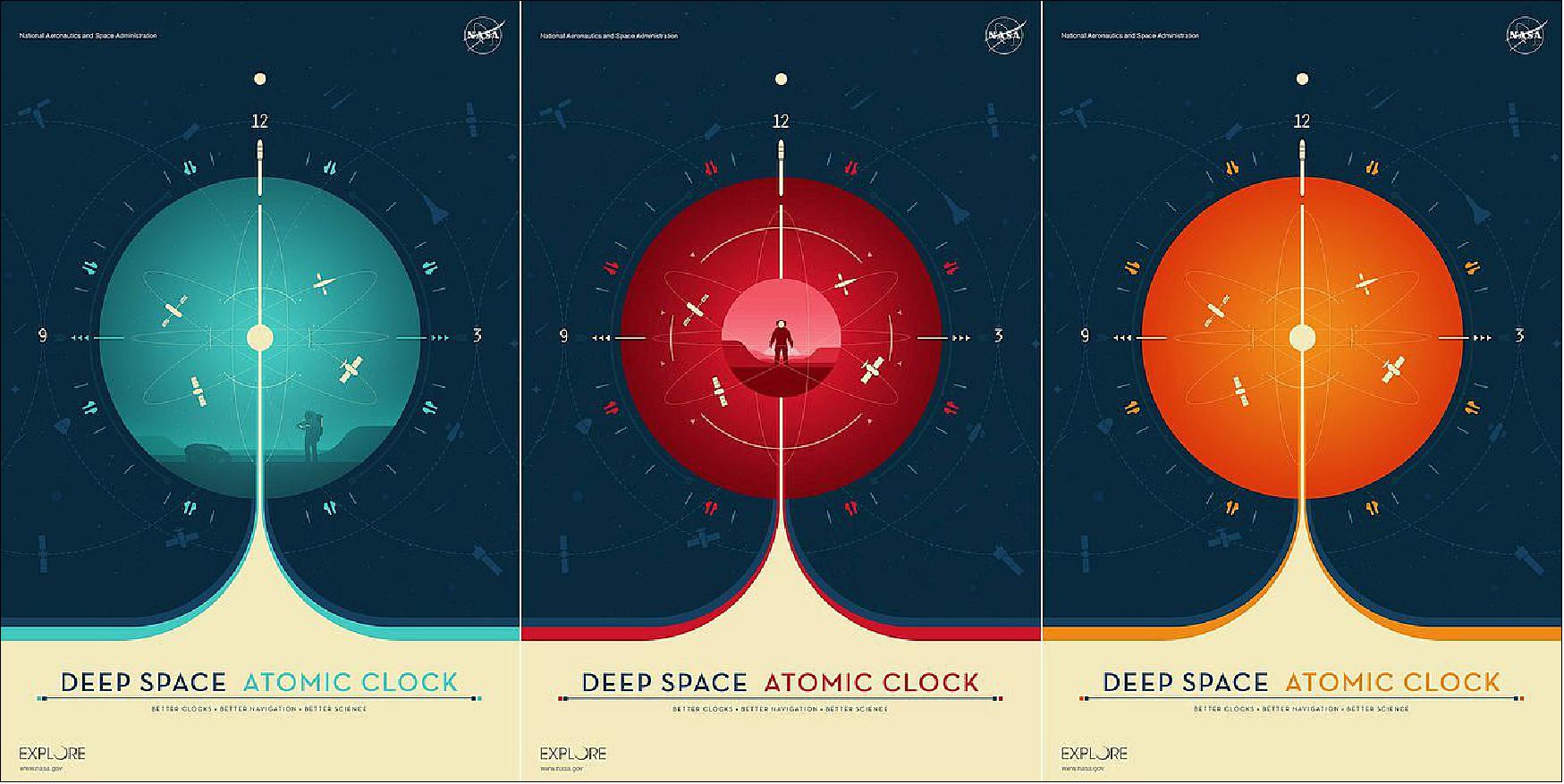
- In a recent study, the Deep Space Atomic Clock team reported a deviation of less than four nanoseconds after more than 20 days of operation.
- Like its predecessor, the Deep Space Atomic Clock-2 will be a tech demo, meaning that VERITAS will not depend on it to fulfill its goals. But this next iteration will be smaller, use less power, and be designed to support a multi-year mission like VERITAS.
- “It is a remarkable accomplishment by the team – the technology demonstration has proven to be a robust system in orbit, and we are now looking forward to seeing an improved version go to Venus,” said Trudy Kortes, director of technology demonstrations for NASA’s Science and Technology Mission Directorate (STMD) at NASA Headquarters in Washington. “This is what NASA does – we develop new technologies and enhance existing ones to advance human and robotic spaceflight. The Deep Space Atomic Clock truly has the potential to transform how we explore deep space.”
- Jason Mitchell, the director of the Advanced Communications & Navigation Technology Division of NASA’s Space Communications and Navigation (SCaN) at the agency’s headquarters agreed: “The instrument’s performance was truly exceptional and a testament to the capability of the team. Going forward, not only will the Deep Space Atomic Clock enable significant, new operational capabilities for NASA's human and robotic exploration missions, it may also enable deeper exploration of the fundamental physics of relativity, much like the clocks supporting GPS have done.”
- The Deep Space Atomic Clock is hosted on a spacecraft provided by General Atomics Electromagnetic Systems of Englewood, Colorado. It is sponsored by STMD’s Technology Demonstration Missions program located at NASA’s Marshall Space Flight Center in Huntsville, Alabama, and SCaN within NASA’s Human Exploration and Operations Mission Directorate. JPL manages the project.
• June 30, 2021: Designed to improve navigation for robotic explorers and the operation of GPS satellites, the technology demonstration reports a significant milestone. 16)
- Spacecraft that venture beyond our Moon rely on communication with ground stations on Earth to figure out where they are and where they’re going. NASA’s Deep Space Atomic Clock is working toward giving those far-flung explorers more autonomy when navigating. In a new paper published today in the journal Nature, the mission reports progress in their work to improve the ability of space-based atomic clocks to measure time consistently over long periods. 17)
- Known as stability, this feature also impacts the operation of GPS satellites that help people navigate on Earth, so this work also has the potential to increase the autonomy of next-generation GPS spacecraft.
- To calculate the trajectory of a distant spacecraft, engineers send signals from the spacecraft to Earth and back. They use refrigerator-size atomic clocks on the ground to log the timing of those signals, which is essential for precisely measuring the spacecraft’s position. But for robots on Mars or more distant destinations, waiting for the signals to make the trip can quickly add up to tens of minutes or even hours.
- If those spacecraft carried atomic clocks, they could calculate their own position and direction, but the clocks would have to be highly stable. GPS satellites carry atomic clocks to help us get to our destinations on Earth, but those clocks require updates several times a day to maintain the necessary level of stability. Deep space missions would require more stable space-based clocks.
- Managed by NASA/JPL, the Deep Space Atomic Clock has been operating aboard General Atomic’s Orbital Test Bed spacecraft since June 2019. The new study reports that the mission team has set a new record for long-term atomic clock stability in space, reaching more than 10 times the stability of current space-based atomic clocks, including those on GPS satellites.
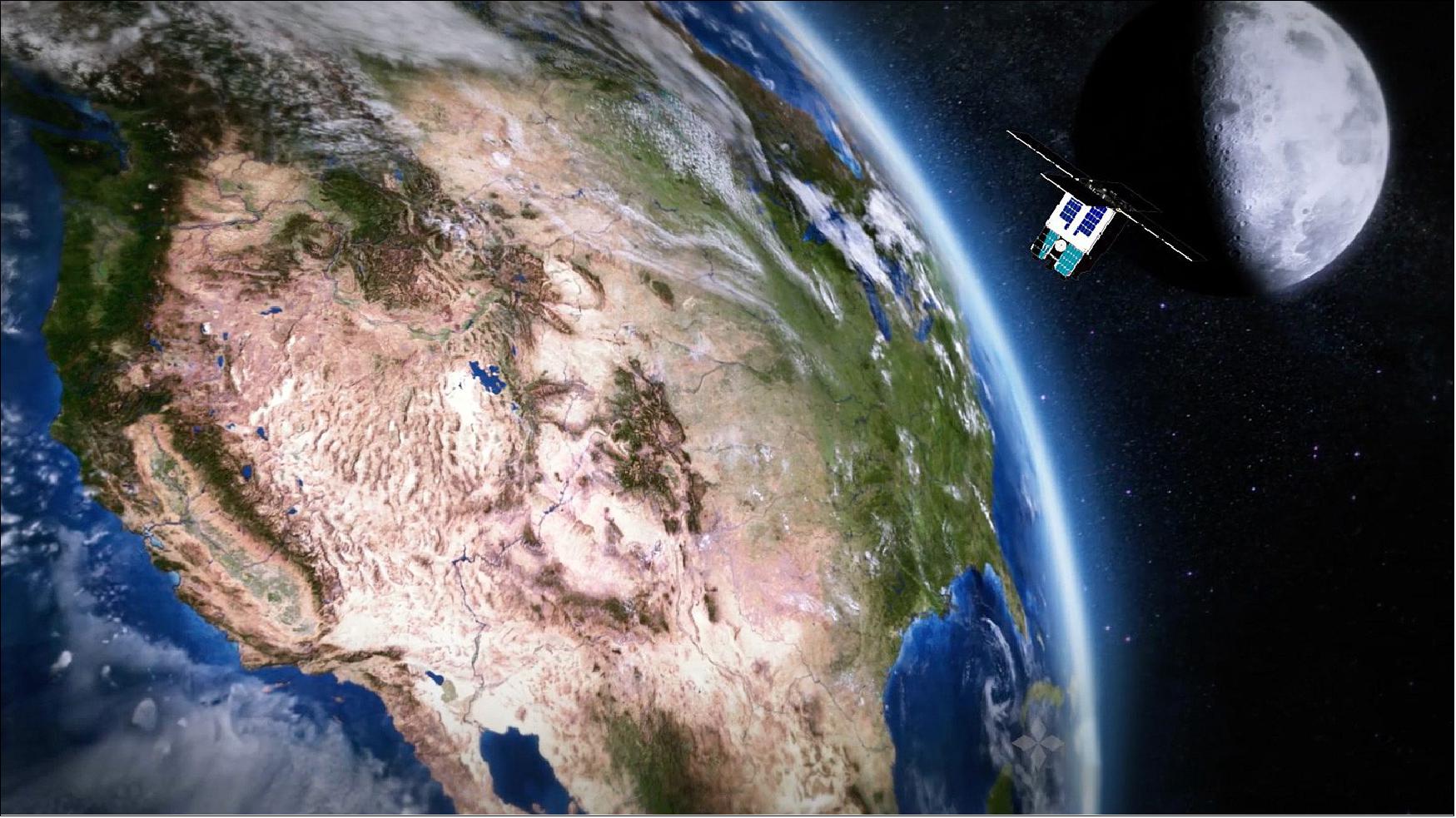
When Every Nanosecond Counts
- All atomic clocks have some degree of instability that leads to an offset in the clock’s time versus the actual time. If not corrected, the offset, while miniscule, increases rapidly, and with spacecraft navigation, even a tiny offset could have drastic effects.
- One of the key goals of the Deep Space Atomic Clock mission was to measure the clock’s stability over longer and longer periods, to see how it changes with time. In the new paper, the team reports a level of stability that leads to a time deviation of less than four nanoseconds after more than 20 days of operation.
- “As a general rule, an uncertainty of one nanosecond in time corresponds to a distance uncertainty of about one foot,” said Eric Burt, an atomic clock physicist for the mission at JPL and co-author of the new paper. “Some GPS clocks must be updated several times a day to maintain this level of stability, and that means GPS is highly dependent on communication with the ground. The Deep Space Atomic Clock pushes this out to a week or more, thus potentially giving an application like GPS much more autonomy.”
- The stability and subsequent time delay reported in the new paper is about five times better than what the team reported in the spring of 2020. This does not represent an improvement in the clock itself, but in the team’s measurement of the clock’s stability. Longer operating periods and almost a full year of additional data made it possible to improve the precision of their measurement.
- The Deep Space Atomic Clock mission will conclude in August, but NASA announced that work on this technology continues: the Deep Space Atomic Clock-2, an improved version of the cutting-edge timekeeper, will fly on the VERITAS (short for Venus Emissivity, Radio Science, InSAR, Topography, and Spectroscopy) mission to Venus. Like its predecessor, the new space clock is a technology demonstration, meaning its goal is to advance in-space capabilities by developing instruments, hardware, software, or the like that doesn't currently exist. Built by JPL and funded by NASA’s Space Technology Mission Directorate (STMD), the ultra-precise clock signal generated with this technology could help enable autonomous spacecraft navigation and enhance radio science observations on future missions.
- “NASA’s selection of Deep Space Atomic Clock-2 on VERITAS speaks to this technology’s promise,” said Todd Ely, Deep Space Atomic Clock principal investigator and project manager at JPL. “On VERITAS, we aim to put this next generation space clock through its paces and demonstrate its potential for deep space navigation and science.”
- The Deep Space Atomic Clock is hosted on a spacecraft provided by General Atomics Electromagnetic Systems of Englewood, Colorado. It is sponsored by STMD’s Technology Demonstration Missions program located at NASA’s Marshall Space Flight Center in Huntsville, Alabama, and NASA’s Space Communications and Navigation (SCaN) program within NASA’s Human Exploration and Operations Mission Directorate. JPL manages the project.
• June 24, 2020: As the time when NASA will begin sending humans back to the Moon draws closer, crewed trips to Mars are an enticing next step. But future space explorers will need new tools when traveling to such distant destinations. The DSAC (Deep Space Atomic Clock) mission is testing a new navigation technology that could be used by both human and robotic explorers making their way around the Red Planet and other deep space destinations. 18)
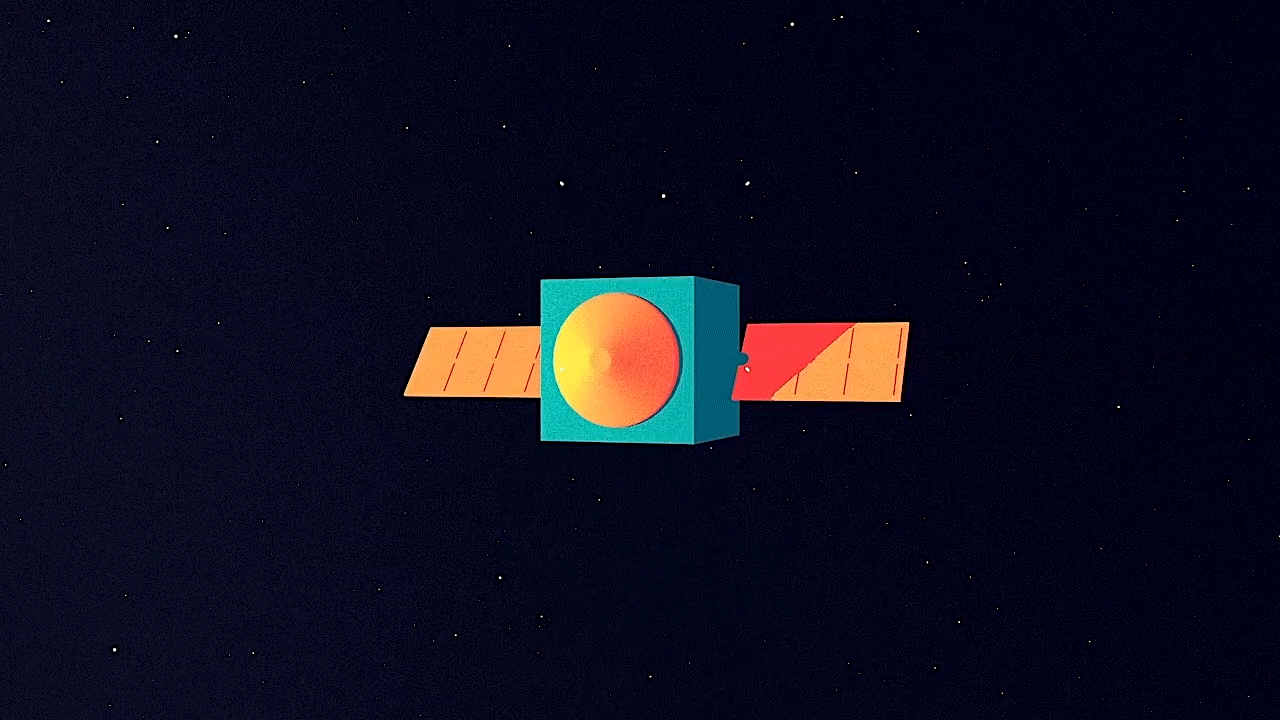
- In less than a year of operations, the mission has passed its primary goal to become one of the most stable clocks to ever fly in space; it is now at least 10 times more stable than atomic clocks flown on GPS satellites. In order to keep testing the system, NASA has extended the mission through August 2021. The team will use the additional mission time to continue to improve the clock's stability, with a goal of becoming 50 times more stable than GPS atomic clocks.
- Launched in June 2019 and managed by NASA's Jet Propulsion Laboratory in Southern California, the toaster-size Deep Space Atomic Clock is a payload on a commercial satellite. As a technology demonstration, its goal is to advance in-space capabilities by developing instruments, hardware, software or the like that doesn't currently exist. These demonstration missions must also show that new technologies can reliably operate in space. The goal is to eventually see such technologies incorporated into full-scale missions.
- In the case of the Deep Space Atomic Clock, the aim is to enable deep space navigation systems that are more autonomous than what exists today. So spacecraft traveling beyond the Moon would have something similar to the GPS-based system that we use on Earth. To do that, the mission is focused on the clock's stability, or its ability to measure time consistently over long periods, while operating in the harsh space environment. The more stable a clock, the longer it can do its job without help from refrigerator-size atomic clocks on the ground.
- "We're extremely proud of what this mission has done already, and we're very excited that NASA thinks it's worthwhile for us to keep working on it," said Todd Ely, Deep Space Atomic Clock principal investigator and project manager at JPL. "This has been an extremely challenging project, but we're motivated by the idea that this technology could fundamentally transform deep space navigation."
• May 11, 2020: The clock is ticking: A technology demonstration that could transform the way humans explore space is active. Developed by NASA’s Jet Propulsion Laboratory in Pasadena, California, the Deep Space Atomic Clock is a serious upgrade to the satellite-based atomic clocks that, for example, enable the GPS on your phone. 19)
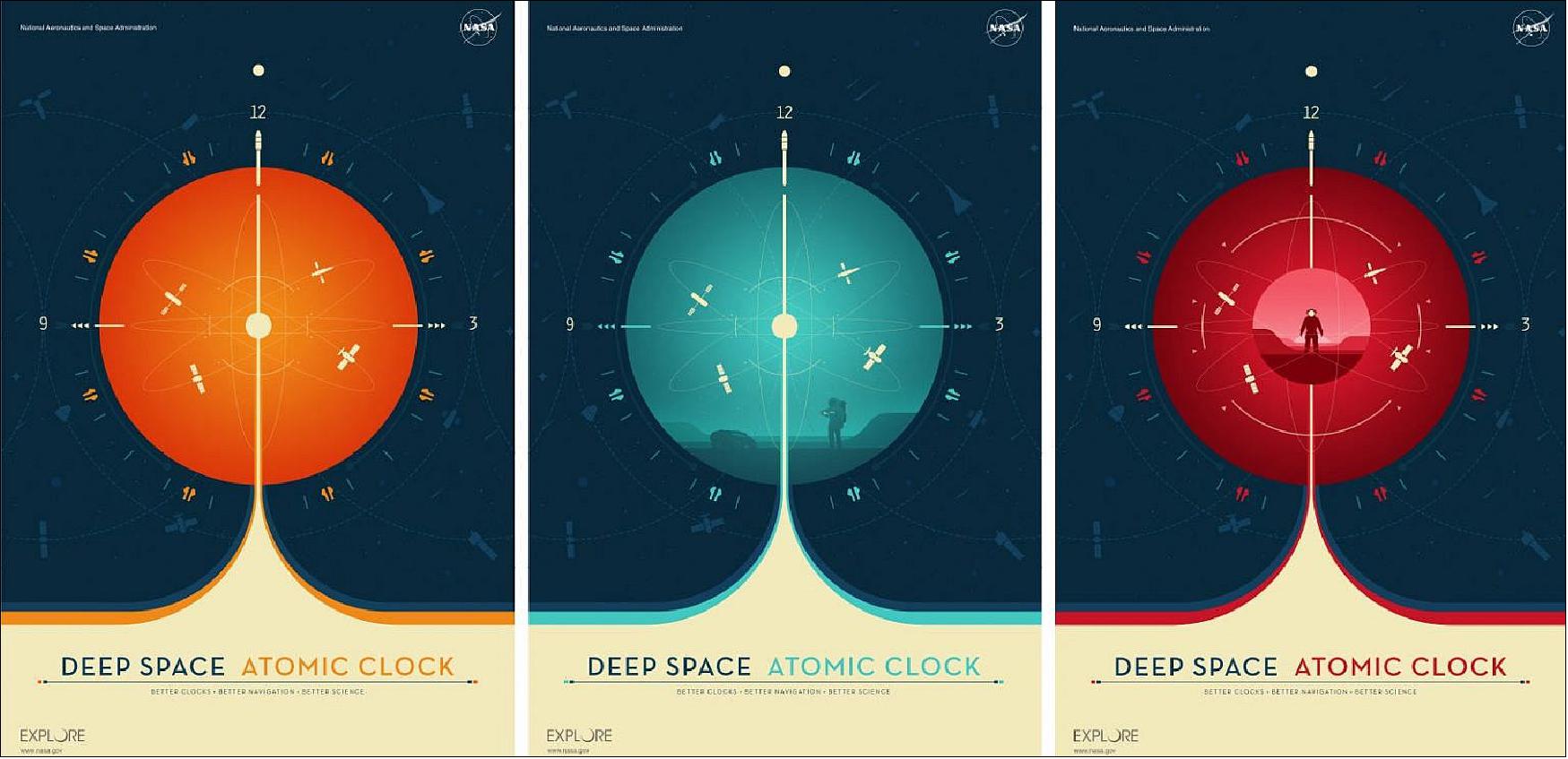
- Ultimately, this new technology could make spacecraft navigation to distant locations like Mars more autonomous. But what is an atomic clock? How are they used in space navigation, and what makes the Deep Space Atomic Clock different? Read on to get all the answers.
Why do we use clocks to navigate in space?
- To determine a spacecraft’s distance from Earth, navigators send a signal to the spacecraft, which then returns it to Earth. The time the signal requires to make that two-way journey reveals the spacecraft’s distance from Earth, because the signal travels at a known speed (the speed of light).
- While it may sound complicated, most of us use this concept every day. The grocery store might be a 30-minute walk from your house. If you know you can walk about a mile in 20 minutes, then you can calculate the distance to the store.
- By sending multiple signals and taking many measurements over time, navigators can calculate a spacecraft’s trajectory: where it is and where it’s headed.
- Most modern clocks, from wristwatches to those used on satellites, keep time using a quartz crystal oscillator. These devices take advantage of the fact that quartz crystals vibrate at a precise frequency when voltage is applied to them. The vibrations of the crystal act like the pendulum of a grandfather clock, ticking off how much time has passed.
- To know the spacecraft’s position within a meter, navigators need clocks with precision time resolution — clocks that can measure billionths of a second (< 10-9 s).
- Navigators also need clocks that are extremely stable. “Stability” refers to how consistently a clock measures a unit of time; its measurement of the length of a second, for example, needs to be the same (to better than a billionth of a second) over days and weeks.
What do atoms have to do with clocks?
- By space navigation standards, quartz crystal clocks aren’t very stable. After only an hour, even the best-performing quartz oscillators can be off by a nanosecond (one billionth of a second). After six weeks, they may be off by a full millisecond (one thousandth of a second), or a distance error of 185 miles (300 km). That would have a huge impact on measuring the position of a fast-moving spacecraft.
- Atomic clocks combine a quartz crystal oscillator with an ensemble of atoms to achieve greater stability. NASA’s DSAC (Deep Space Atomic Clock) will be off by less than a nanosecond after four days and less than a microsecond (one millionth of a second) after 10 years. This is equivalent to being off by only one second every 10 million years.

- Atoms are composed of a nucleus (consisting of protons and neutrons) surrounded by electrons. Each element on the periodic table represents an atom with a certain number of protons in its nucleus. The number of electrons swarming around the nucleus can vary, but they must occupy discreet energy levels, or orbits.
- A jolt of energy — in the form of microwaves — can cause an electron to rise to a higher orbit around the nucleus. The electron must receive exactly the right amount of energy — meaning the microwaves must have a very specific frequency — in order to make this jump.
- The energy required to make electrons change orbits is unique in each element and consistent throughout the universe for all atoms of a given element. For instance, the frequency necessary to make electrons in a carbon atom change energy levels is the same for every carbon atom in the universe. The Deep Space Atomic Clock uses mercury atoms; a different frequency is necessary to make those electrons change levels, and that frequency will be consistent for all mercury atoms.
- “The fact that the energy difference between these orbits is such a precise and stable value is really the key ingredient for atomic clocks,” said Eric Burt, an atomic clock physicist at JPL. “It’s the reason atomic clocks can reach a performance level beyond mechanical clocks.”
- Being able to measure this unchangeable frequency in a particular atom offers science a universal, standardized measurement of time. (“Frequency” refers to the number of waves that pass a particular point in space in a given unit of time. So, by counting waves, it’s possible to measure time.) In fact, the official measurement of the length of a second is determined by the frequency needed to make electrons jump between two specific energy levels in a cesium atom.
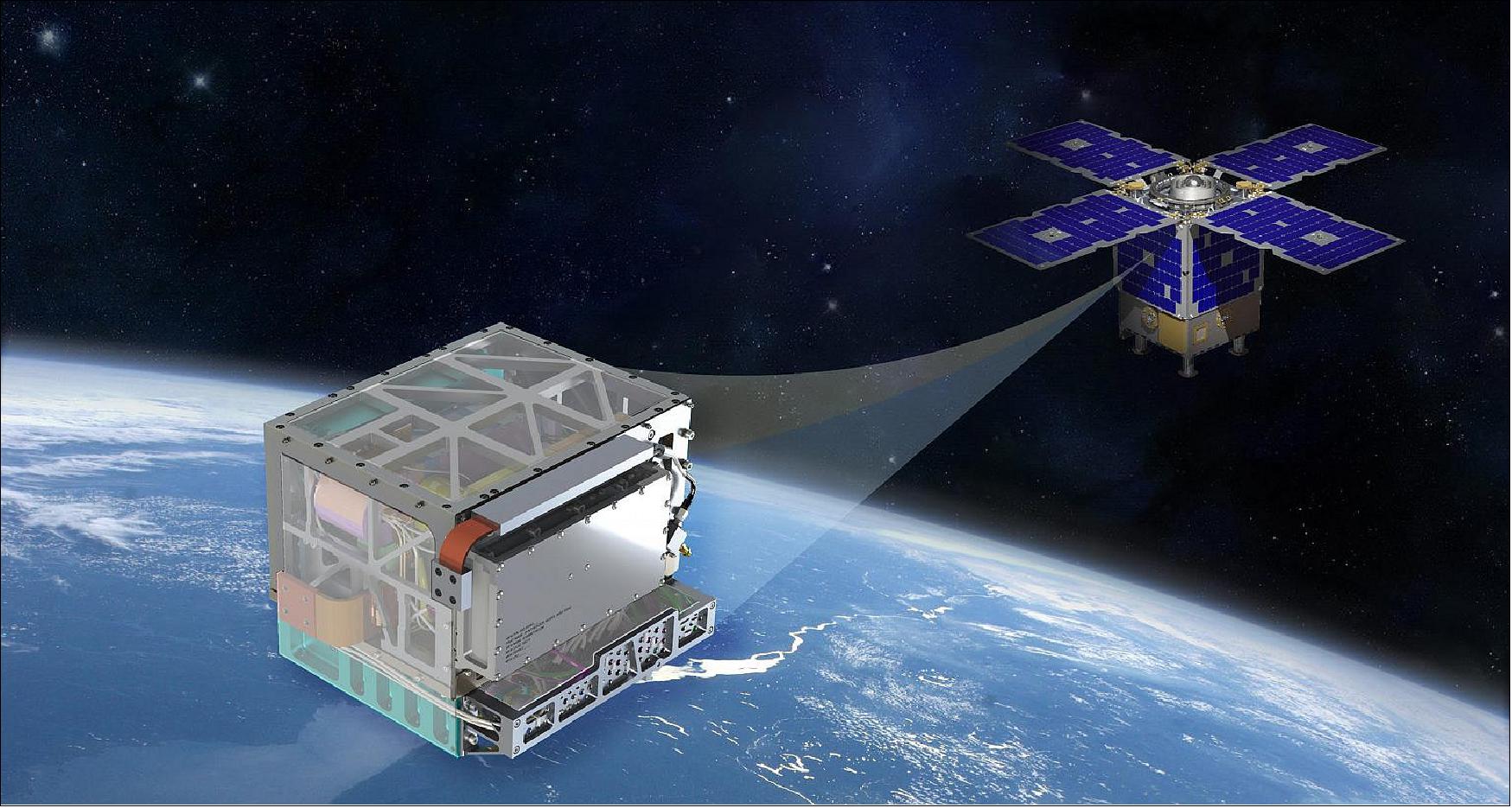
- In an atomic clock, the frequency of the quartz oscillator is transformed into a frequency that is applied to a collection of atoms. If the derived frequency is correct, it will cause many electrons in the atoms to change energy levels. If the frequency is incorrect, far fewer electrons will jump. This will determine if the quartz oscillator is off-frequency and by how much. A “correction” determined by the atoms can then be applied to the quartz oscillator to steer it back to the correct frequency. This type of correction is calculated and applied to the quartz oscillator every few seconds in the Deep Space Atomic Clock.
What’s unique about the Deep Space Atomic Clock?
- Atomic clocks are used onboard GPS satellites that orbit the Earth, but even they must be sent updates two times per day to correct the clocks’ natural drift. Those updates come from more stable atomic clocks on the ground that are large (often the size of a refrigerator) and not designed to survive the physical demands of going to space.
- Up to 50 times more stable than the atomic clocks on GPS satellites, NASA’s Deep Space Atomic Clock is intended to be the most stable atomic clock ever flown in space. It achieves this stability by using mercury ions.
- Ions are atoms that have a net electric charge, rather than being electrically neutral. In any atomic clock, the atoms are contained in a vacuum chamber, and in some of those clocks, atoms interact with the vacuum chamber walls. Environmental changes such as temperature will then cause similar changes in the atoms and lead to frequency errors. Many atomic clocks use neutral atoms, but because the mercury ions have an electric charge, they can be contained in an electromagnetic “trap” to prevent this interaction from happening, allowing the Deep Space Atomic Clock to achieve a new level of precision.
- For missions going to distant destinations like Mars or other planets, such precision makes autonomous navigation possible with minimal communication to and from Earth — a huge improvement in how spacecraft are currently navigated.
• December 20, 2019: General Atomics Electromagnetic Systems (GA-EMS) announced that in early November NASA ended the commissioning phase of the Deep Space Atomic Clock (DSAC), the primary payload on-board GA-EMS’ Orbital Test Bed (OTB) satellite, with DSAC now in nominal operation to demonstrate its capabilities supporting deep space navigation and exploration. OTB was launched from Cape Canaveral on June 25, 2019 on a SpaceX Falcon Heavy rocket. 20)
- “OTB continues to operate nominally, and we are excited that DSAC is performing as anticipated,” stated Scott Forney, president of GA-EMS. “We continue to provide operation services to the Jet Propulsion Laboratory (JPL) and NASA for the currently scheduled year-long trial of DSAC’s functionality and utility for one-way-based space navigation. We are very excited to be supporting a project that has the potential to change the future of deep space navigation.”
- DSAC is a miniaturized, ultra-precise, mercury-ion atomic clock designed and built at NASA’s JPL for NASA Space Technology Mission Directorate’s Technology Demonstration Missions Program. DSAC provides extreme accuracy and greater stability than today’s best navigation clocks to support exploration deeper into the solar system, as well as potentially provide benefits to Earth users worldwide.
- “In addition to DSAC, commissioning of the other demonstration payloads on-board OTB continues according to each customer’s schedule,” stated Nick Bucci, vice president of Missile Defense and Space Systems at GA-EMS. “We anticipate OTB to remain mission capable for up to seven years, providing customers with a significant timeframe to perform and complete their individual mission objectives.”
- GA-EMS’ OTB is a configurable, versatile platform designed to space-qualify multiple payloads on a single satellite. OTB increases the number of flight opportunities for customers looking to launch their payload into orbit without bearing the costly burden of designing, building and launching a dedicated spacecraft. GA-EMS works closely with customers to design and build OTB satellites that simultaneously meet multiple customer mission objectives and requirements. Launch and mission operation services are also provided by GA-EMS to support the full mission lifecycle for each customer payload.
• August 26, 2019: An atomic clock that could pave the way for autonomous deep space travel was successfully activated last week and is ready to begin its year-long tech demo, the mission team confirmed on Friday, Aug. 23, 2019. Launched in June, NASA's Deep Space Atomic Clock (DSAC) is a critical step toward enabling spacecraft to safely navigate themselves in deep space rather than rely on the time-consuming process of receiving directions from Earth. 21)
- Developed at NASA's Jet Propulsion Laboratory in Pasadena, California, the clock is the first timekeeper stable enough to map a spacecraft's trajectory in deep space while being small enough to fly onboard the spacecraft. A more stable clock can operate farther from Earth, where it needs to work well for longer periods than satellites closer to home.
- Atomic clocks, like those used in GPS satellites, are used to measure the distance between objects by timing how long it takes a signal to travel from Point A to Point B. For space exploration, atomic clocks must be extremely precise: an error of even one second means the difference between landing on a planet like Mars or missing it by hundreds of thousands of miles. Up to 50 times more stable than the atomic clocks on GPS satellites, the mercury-ion Deep Space Atomic Clock loses one second every 10 million years, as proven in controlled tests on Earth. Now it will test that accuracy in space.
- Navigators currently use refrigerator-size atomic clocks on Earth to pinpoint a spacecraft's location. Minutes to hours can go by as a signal is sent from Earth to the spacecraft before being returned to Earth, where it is used to create instructions that are then sent back to the spacecraft. A clock aboard a spacecraft would allow the spacecraft to calculate its own trajectory, instead of waiting for navigators on Earth to send that information. This advancement would free missions to travel farther and, eventually, carry humans safely to other planets.
- "The goal of the space experiment is to put the Deep Space Atomic Clock in the context of an operating spacecraft - complete with the things that affect the stability and accuracy of a clock - and see if it performs at the level we think it will: with orders of magnitude more stability than existing space clocks," said navigator Todd Ely, principal investigator of the project at JPL.
- In coming months, the team will measure how well the clock keeps time down to the nanosecond. The results begin the countdown to a day when technology can safely help astronauts navigate themselves to other worlds.
- The Deep Space Atomic Clock is hosted on a spacecraft provided by General Atomics Electromagnetic Systems of Englewood, Colorado. It is sponsored by the Technology Demonstration Missions program within NASA's Space Technology Mission Directorate and the Space Communications and Navigations program within NASA's Human Exploration and Operations Mission Directorate. JPL manages the project.
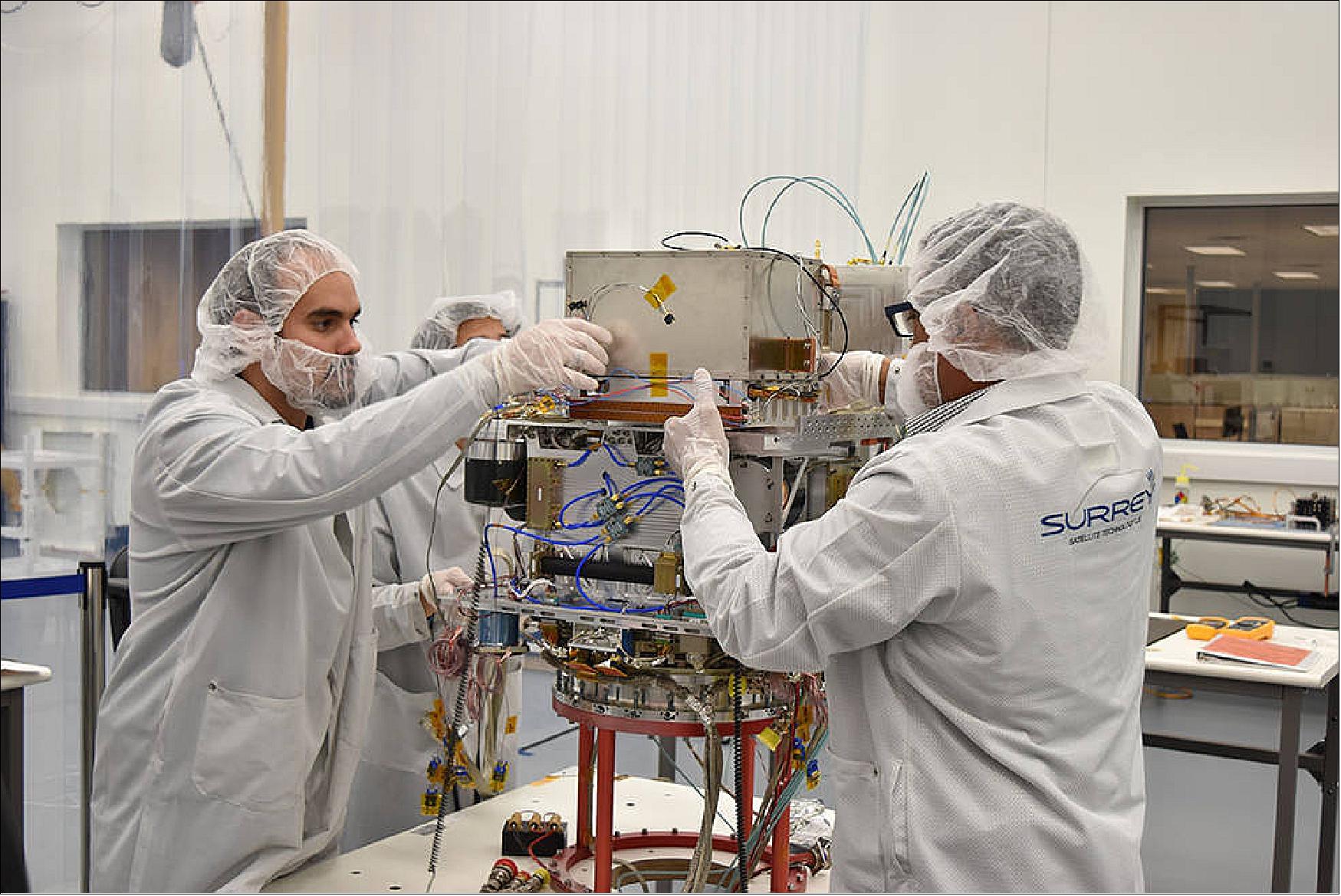
• July 23, 2019: Viasat Inc., a global communications company, announced today its Real-Time Earth (RTE) service achieved a major milestone by providing ground station service support to General Atomics Electromagnetic Systems' (GA-EMS) Orbital Test Bed (OTB) satellite after its successful launch on a SpaceX Falcon Heavy rocket on June 25, 2019. Once in orbit, Viasat's RTE service provided critical satellite commissioning activities and subsequent operations from two of its U.S. ground stations: one in Georgia and the other in Hawaii. 22)
- The GA-EMS OTB-1 satellite is a new paradigm for LEO (Low Earth Orbit) hosted payload spacecraft, and provides customers with affordable access to space in order to test and qualify various technologies. Viasat's role in the OTB program has been to provide initial communications, control and telemetry services to the spacecraft via the Viasat RTE ground station network.
- Viasat's RTE network provides Ground-Station-as-a-Service (GSaaS) to the Earth Observation and remote sensing community. The service offers affordability and reduced latency through automation and geographic diversity on a pay-per-use basis. Viasat's RTE service can support next-generation and legacy LEO satellites using the S-, X-, and Ka-bands, which enables operators to meet today's and tomorrow's data requirements.
- "Supporting General Atomics Electromagnetic Systems in the successful launch and early orbit operations of the first OTB satellite is a testament to the strength of our RTE ground-to-space tracking services," said John Williams, vice president for Real-Time Earth services at Viasat. "As our RTE network grows in terms of locations and capabilities, we feel confident we can provide enhanced state-of-the-art GSaaS capabilities and expect to grow our customer base and opportunities, globally."
- Viasat is a global communications company that believes everyone and everything in the world can be connected. For more than 30 years, Viasat has helped shape how consumers, businesses, governments and militaries around the world communicate. Today, the Company is developing the ultimate global communications network to power high-quality, secure, affordable, fast connections to impact people's lives anywhere they are—on the ground, in the air or at sea.
Sensor Complement
In addition to DSAC, OTB-1 will carry a Surrey payload suite comprised of the Electronics Test Bed, the FlexRX receiver, and the RadMon sensor. Developed by Surrey University in the UK, the Electronics Test Bed will evaluate and demonstrate a range of new electronic components, processors, and memory devices, enabling in-orbit heritage to be gained on components that may be incorporated into future designs. 23)
DSAC (Deep Space Atomic Clock)
Some background: In 2012, DSAC is a NASA technology demonstration mission with the goal to validate a miniaturized, ultra-precise mercury-ion atomic clock that is 100 times more stable than today’s best navigation clocks. The DSAC project is sponsored by the NASA Space Technology Mission Directorate and managed by NASA(JPL (Jet Propulsion Laboratory) in Pasadena, CA.
On June 27, 2013, NASA/JPL selected SST-US LLC for the flight of the DSAC (Deep Space Atomic Clock) payload under the sponsorship of NASA's STMD (Space Technology Mission Directorate). DSAC will fly on the SST-US-owned-and-operated OTB (Orbital Test Bed) satellite. 24)
Over the past 20 years, NASA/JPL engineers have been steadily improving and miniaturizing the mercury-ion trap atomic clock, preparing it to operate in the harsh environment of deep space. In the laboratory setting, the Deep Space Atomic Clock's precision has been refined to permit drift of no more than 1 nanosecond in 10 days. 25) 26) 27) 28) 29)
Benefits of the Clock to Navigation and Science: In today’s 2-way navigation architecture, the Earth ground network tracks a user spacecraft and then a ground-based team performs navigation. Relative to this, a 1-way navigation architecture can deliver more data with better accuracy, and is enabling for future autonomous radio navigation. Some specific examples of how a 1-Way deep space tracking architecture can benefit NASA include: 30) 31)
4) The DSN can support multiple downlinks on a single antenna, called MSPA (Multiple Spacecraft Per Aperture). Since the DSAC enabled 1-way radiometric tracking doesn’t require an uplink, it can take full advantage of MSPA. For instance, at Mars two spacecraft equipped with DSAC can be tracked simultaneously on the downlink by a single antenna, while, with the current 2-way tracking capability, those two spacecraft must split their time on the uplink.
5) Deep space users with DSAC can utilize full view periods for tracking, unlike 2-way tracking, which reduces the available tracking time of the view period by the round trip light time. As an example, Cassini’s Northern hemisphere view periods at Goldstone and Madrid are on the order of 11 hrs, so a round trip light time in the 4 – 5 hr range yields an effective ~ 6 hr 2-way pass. A 1-way pass using DSAC can utilize the full view period of 11 hrs, a near doubling of the usable data without needing to transition into a complicated 3-way tracking operation across multiple ground stations.
6) For outer planet missions, solar corona plasma effects are a frequency-dependent error source that dominates over other measurement errors and affects radiometric tracking across both short and long time scales. Use of a Ka-only 1-way downlink reduces these effects by 10 times relative to the typical user on an X-up/Ka-down 2-way paradigm. Indeed the Europa Flyby Mission’s gravity science results needed for determining the presence of an ocean underneath the crustal ice requires Ka-band tracking, which is enabled via having DSAC.
7) Planetary atmosphere investigations using radio occultations can benefit from DSAC as well. Compared to today’s radio occultations that rely on 1-way tracking derived using ultra stable oscillators, DSAC enabled measurements are upwards of 10 times more accurate on the time scales relevant to these experiments (that is, the several minutes that a spacecraft radio signal to Earth rises and sets as it passes through the atmosphere of interest before being occulted by the planet).
8) A 1-way uplink received by a DSAC-enabled spacecraft with a properly configured and capable on-board navigation system is able to self-navigate in deep space. Autonomous deep space navigation has been demonstrated using optical navigation with the DS1 (Deep Space 1) and Deep Impact missions. However, a complete implementation of a fully autonomous on-board navigation system would couple a DSAC-enabled 1-way forward radiometric tracking system with optical tracking from a camera system. This would combine the strengths of radio navigation for determining absolute location in deep space and in planetary orbit with the target relative navigation provided by the optical system. A combined 1-way radiometric and optical autonomous navigation system would provide a powerful solution for robotic missions where ground-in-the-loop operations are impossible (deep space encounters, planetary capture, real-time orbital operations, etc.), as well as supporting human exploration missions beyond LEO (Low Earth Orbit) that require crewed operations without ground support.
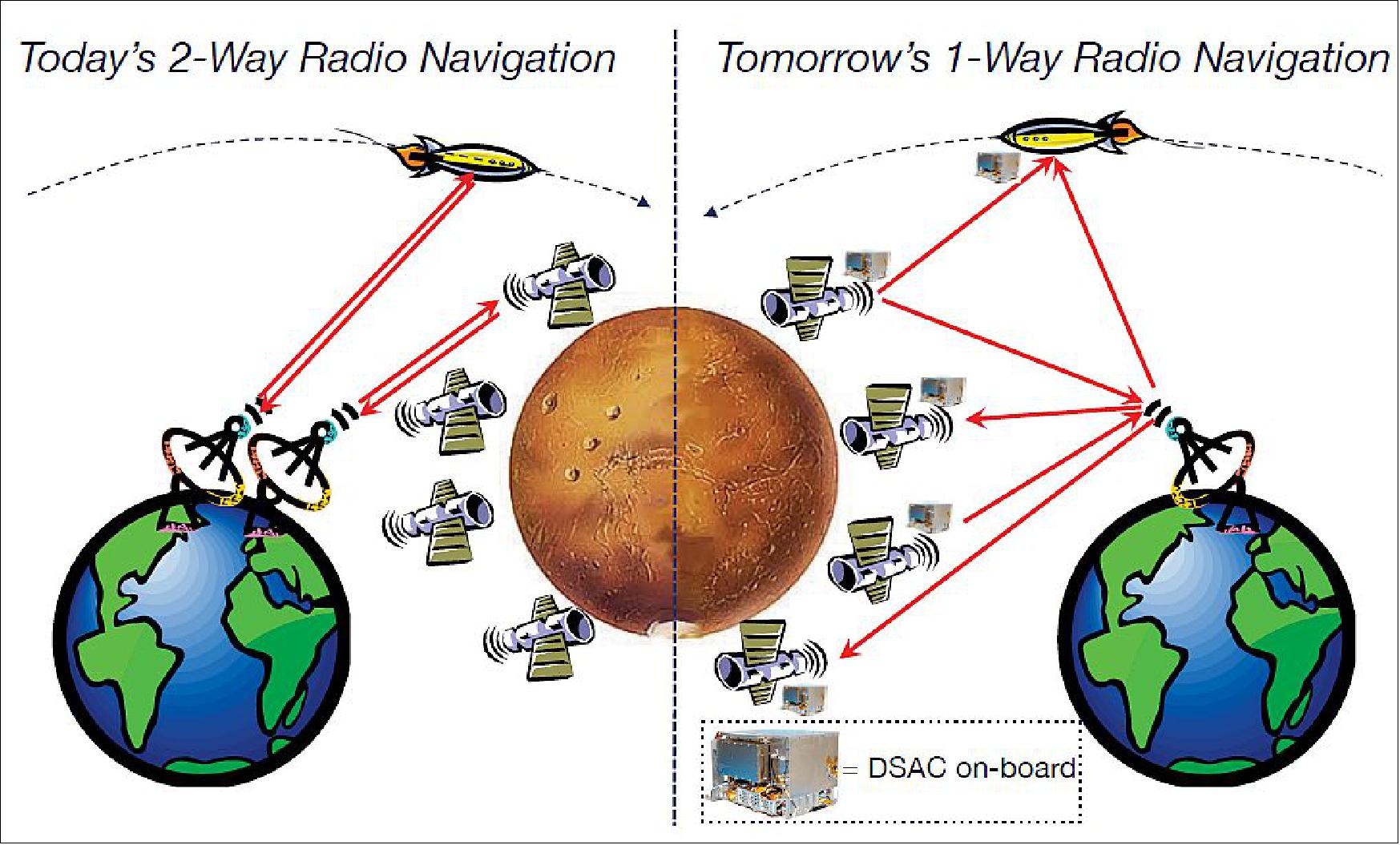
Once in LEO, DSAC’s spaceborne performance will be characterized via a year-long demonstration.
DSAC Instrument Overview
The DSAC project will advance the 199Hg+ atomic space clock technology from a TRL (Technology Readiness Level) 5 to TRL 7 by developing an advanced prototype, verifying its performance in space, and demonstrating its viability as navigation hardware. The DSAC technology uses the property of mercury ions’ hyperfine transition frequency at 40.50 GHz to effectively “steer” the frequency output of a quartz oscillator to a near-constant value. DSAC does this by confining the mercury ions with electric fields in a trap (Figure 1) and protecting them by applying magnetic fields and shielding. This provides a stable environment for measuring the hyperfine transition very accurately and minimizes sensitivity to temperature and magnetic variations. Coupling this with the fact that the DSAC technology has almost no expendables enables development of a clock suitable for very long-duration space missions. 32)
The current best estimate (December 2013) of the DSAC Demonstration Unit’s (DU) size, mass, and average power are: 29 cm x 26 cm x 23 cm, 17.5 kg, and 44 W, respectively. The preliminary configuration of the DU is shown in Figures 1 and 23. Another project objective is to identify improvements needed for a flight version of DSAC so that it can be readily used by a future deep space mission or GNSS application. In particular, DSAC elements amenable to reductions in size, mass, and power.
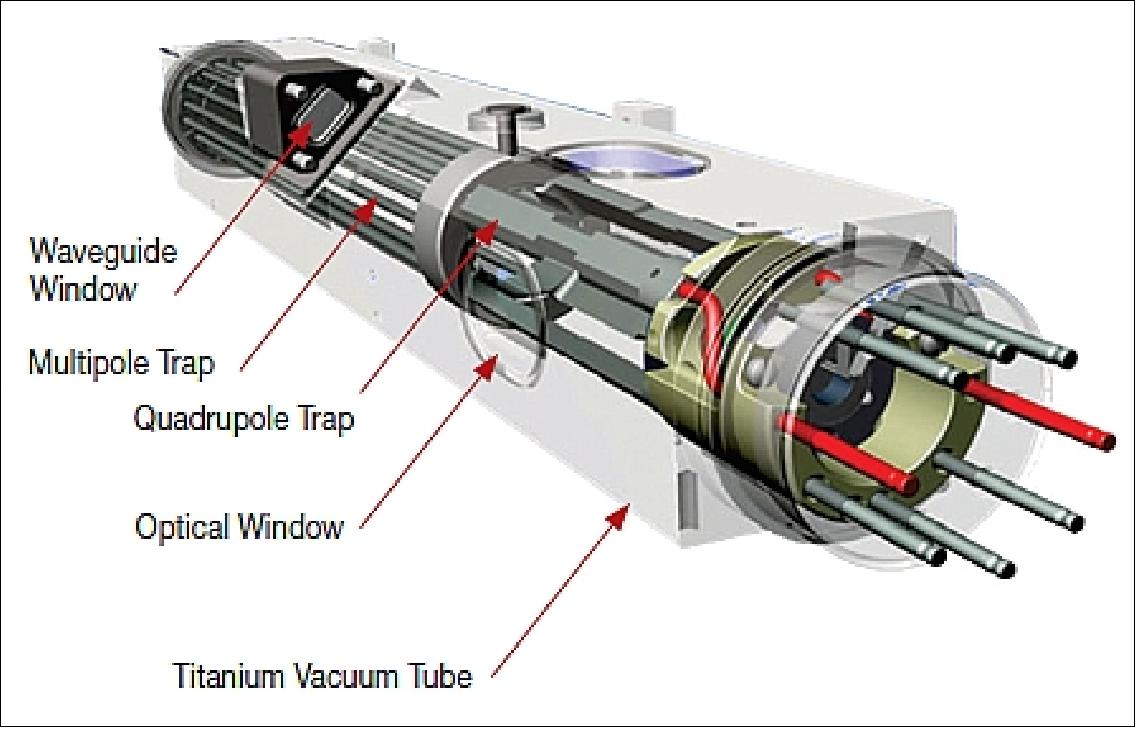
Legend to Figure 22: The quadrupole trap is where the hyperfine transition is optically measured and the multipole trap is where the ions are “interrogated” by a microwave signal via a waveguide from the quartz oscillator.
Ground-based atomic clocks are the cornerstone of spacecraft navigation for most deep space missions because of their use in forming precision two-way coherent Doppler and range measurements. DSAC will deliver the same stability and accuracy for spacecraft exploring the solar system by providing an equivalent capability on-board a spacecraft for forming precision one-way radiometric tracking data (i.e., range, Doppler, and phase). This new capability could forever change the way we conduct deep-space navigation — by eliminating the need to "turn signals around" for tracking. Much the same way modern GPS (Global Positioning Systems) use one-way signals to enable terrestrial navigation services, the DSAC will provide the same capability in deep-space navigation — with such extreme accuracy that researchers will be required to carefully account for the effects of relativity, or the relative motion of an observer and observed objected, as impacted by gravity, space and time (clocks in GPS-based satellite, for example, must be corrected to account for this effect, or their navigational fixes begin to drift). 33)
DSAC’s stability, as measured by its AD (Allan Deviation), is expected to be less than 3 x 10-15 at one day, with a ground laboratory version of DSAC currently demonstrating an AD of approximately 1 x 10 -15 at one day. Such a small spacecraft clock error will enable one-way radiometric tracking data with accuracy equivalent to or better than current two-way tracking data, allowing a shift to a more efficient and flexible one-way deep space navigation architecture.
The DSAC team at JPL is preparing a miniaturized, low-mass atomic clock — orders of magnitude smaller, lighter and more stable than any other atomic clock flown in space — for a test flight in LEO (Low Earth Orbit). The clock will make use of GPS signals to demonstrate precision orbit determination and confirm its performance, promising new savings on mission operations costs, delivering more science data and enabling further development of deep-space autonomous radio navigation.
The DSAC demonstration unit (Figure 23) and payload will be hosted on a spacecraft provided by Surrey Satellite Technologies US LLC, Englewood, CO. It will launch in 2015 into Earth orbit. The DSAC payload will be operated for at least a year to demonstrate its functionality and utility for one-way-based navigation.
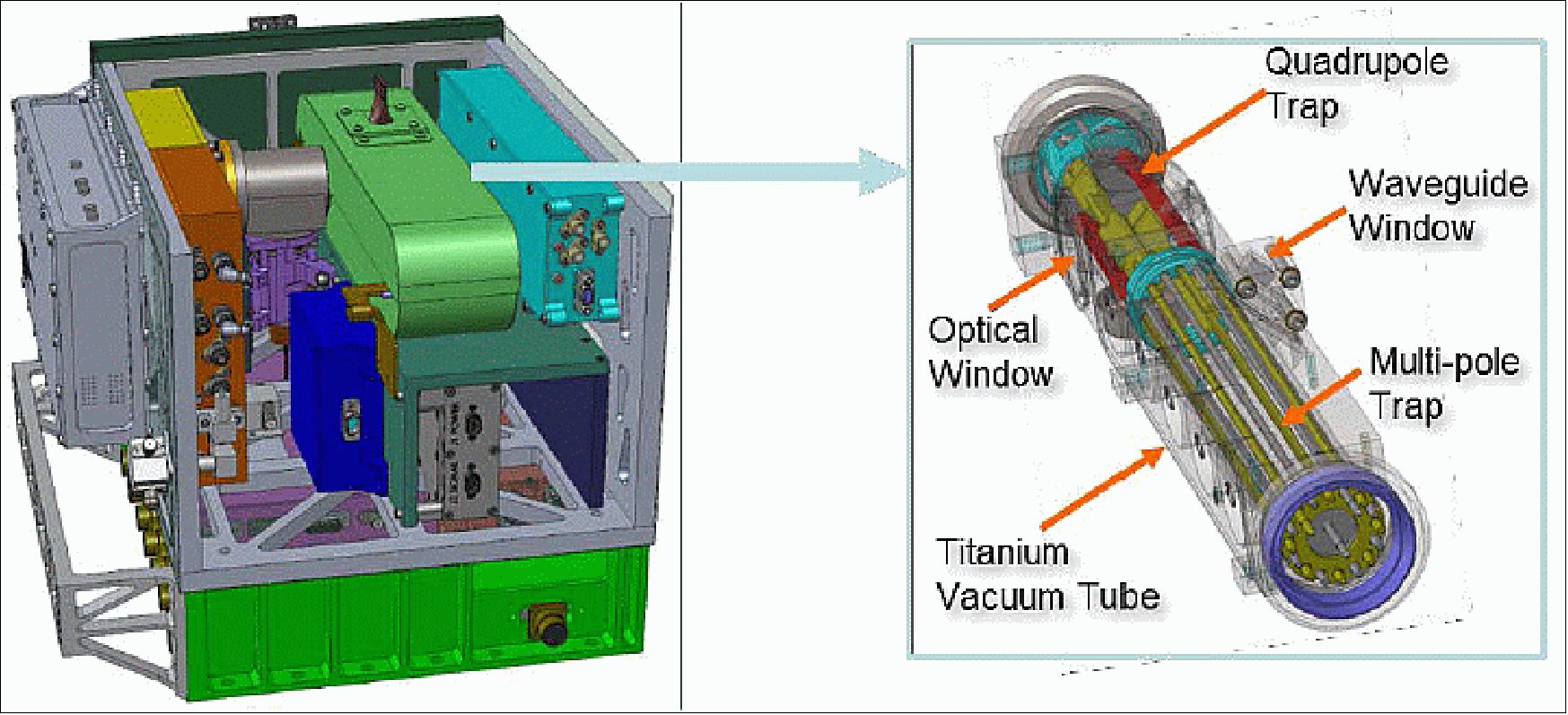
The mission requirement is to validate the clock’s stability and drift with an AD of at most 2.0 x 10-14 at one day while in orbit. This requirement is looser than the expected performance of the clock because of the presence of systematic errors in the measurement system; however, the performance of the validation system (like the clock itself) is expected to be better than this required level.
The DSAC payload consists of three subsystems (and associated cabling):
• An ovenized crystal USO (Ultra Stable Oscillator) produced by FEI (Frequency Electronics Incorporated) with short term (between 1 – 100 seconds) stability of at most 2 x 10 -13 and frequency drift below 1 x 10 -10/day
• The DSAC DU (Demonstration Unit)
• The GPS system is comprised of a JPL-designed TriG-POD receiver, produced by Moog Broad Reach, and a zenith-pointing choke ring antenna, designed to minimize multipath effects. The TriG receiver is designed to autonomously collect L1, L2, and L5 (not used by DSAC) carrier phase and pseudorange data from up to 24 in-view GPS (Global Positioning System) satellites or other GNSS satellites (also not currently used/needed by DSAC).
Additionally, the DSAC flight software is resident on the OTB’s Payload Interface Unit. The payload functions via the USO providing the input frequency to the DU. The DU calculates corrections to the USO signal to produce a stabilized frequency output. This output signal is then used as the reference for the GPS receiver. The GPS system collects the carrier phase and pseudorange data from the GPS constellation (Figure 5) that is later telemetered to the ground for use in the precision clock determination process. The DSAC payload of the USO, DU, and GPS receiver reside in the payload bay of the spacecraft located in the mid-section of the spacecraft bus. The GPS antenna resides on the space-facing panel of the spacecraft, in a configuration that provides an unobstructed view of the GPS constellation during the nominal mission (after launch and early operations has established the nominal attitude). This enables continuous GPS data collection throughout the mission, excepting GPS receiver resets.
Nominal Mission Operations of DSAC
The DSAC investigation team consists of two main teams, the TDAS (Technology Demonstration Analysis System ) team (or analysis team for short) and the payload operations team. The TDAS team is responsible for analysis of the collected GPS data to determine the long-term stability of DSAC. GPS orbit and clock solutions are collected by the TDAS system from the JPL GNSS Analysis Center or the IGS (International GNSS Service) via the web. Key TDAS tools used to reconstruct the orbit, relevant dynamic parameters, and the clock performance, include the GNSS-Inferred Positioning System and Orbit Analysis Simulation Software (GIPSY-OASIS) and the Mission-analysis, Operations, and Navigation Toolkit Environment (MONTE). These tools enable the analysis team to perform a combined POD (Precision Orbit Determination) and PCD (Precision Clock Determination) process. The payload operations team is responsible for telemetry collection and commanding, monitoring health and safety, and evaluating the clock technical performance from the telemetry. The telemetry-based assessment will be used in conjunction with the TDAS-based assessment.
Once payload commissioning is complete, the spacecraft is essentially a stable platform for the remainder of the DSAC mission (except for two biannual spacecraft yaw flips). Nominal operations are straightforward with all elements of the DSAC payload powered on, stable, and operating. Data flow to-and-from the payload is depicted in Figure 4. Four types of data are required for the DSAC investigation:
• GPS receiver data (including engineering telemetry and the observables from the GPS constellation) collected nominally on a 10 second interval.
• Clock telemetry (including housekeeping data and clock corrections).
• Platform data, such as attitude, thermal, and other miscellaneous data related to the state of the platform (or externally-sensed temperature of the payload units) that may be useful in correlating signatures in the clock performance to the environment.
• GPS constellation orbit and clock solutions.
The first three data sets are generated on-board and delivered to the DSAC flight team. The GPS constellation orbit and clock solutions used by DSAC are obtained from JPL’s IGS Analysis Center (for JPL orbit and clock solutions) and from NASA’s CDDIS (Crustal Dynamics Data Information System) space geodesy data archive (for IGS orbit and clock solutions). TDAS is responsible for this interface.
The DSAC-specific GPS receiver data and clock telemetry are collected by the on-orbit computer and stored in a file format on-board. At least once daily, these files are made available for downlink via an automated downlink process. Additionally, a set of platform-related data is downlinked (on a schedule defined by the OTB flight team) and made available to the DSAC flight team. This is an automated process that does not require the DSAC flight team to make any specific data request.
After the data is received on the ground, it is placed on a secure FTP server (by the OTB ground system) within two hours of the end of the pass. Nominally, DSAC data is downlinked on one (single) pass per day, where the DSAC data is prioritized over other non-essential data for downlink during that pass. The DSAC data downlink volume is designed to fit within a single nominal pass; however, a latency of three days is possible in the event of lost passes or other anomalies that interfere with the nominal downlink. Approximately five to six downlink passes may be available per day to downlink all of the data from the OTB (including spacecraft data and data from the payloads). The DSAC ground system retrieves the data from the secure FTP server and delivers it to the DSAC local repository.
The secure FTP server is also the DSAC interface to the OTB uplink process. Command request files are generated by the payload operations team in the form of acquisition schedule XML files. The files allow specification of the execution time for each command as an absolute or relative time. Acquisition schedules are placed on the secure FTP server at least 72 hours prior to the required execution time on the spacecraft. The OTB ground system reviews the command requests to confirm they pose no threat to the platform and are consistent with the expected configuration. DSAC is notified within 48 hours of the required execution time that the command is accepted for uplink. The OTB ground system is responsible for generation of the uplink products and getting them on-board in time for their earliest execution time. Once commissioning is complete, very limited commanding is planned (since changes in the on-board state interfere with the long-term Allan deviation determination and no commanding is required for day-to-day operations).
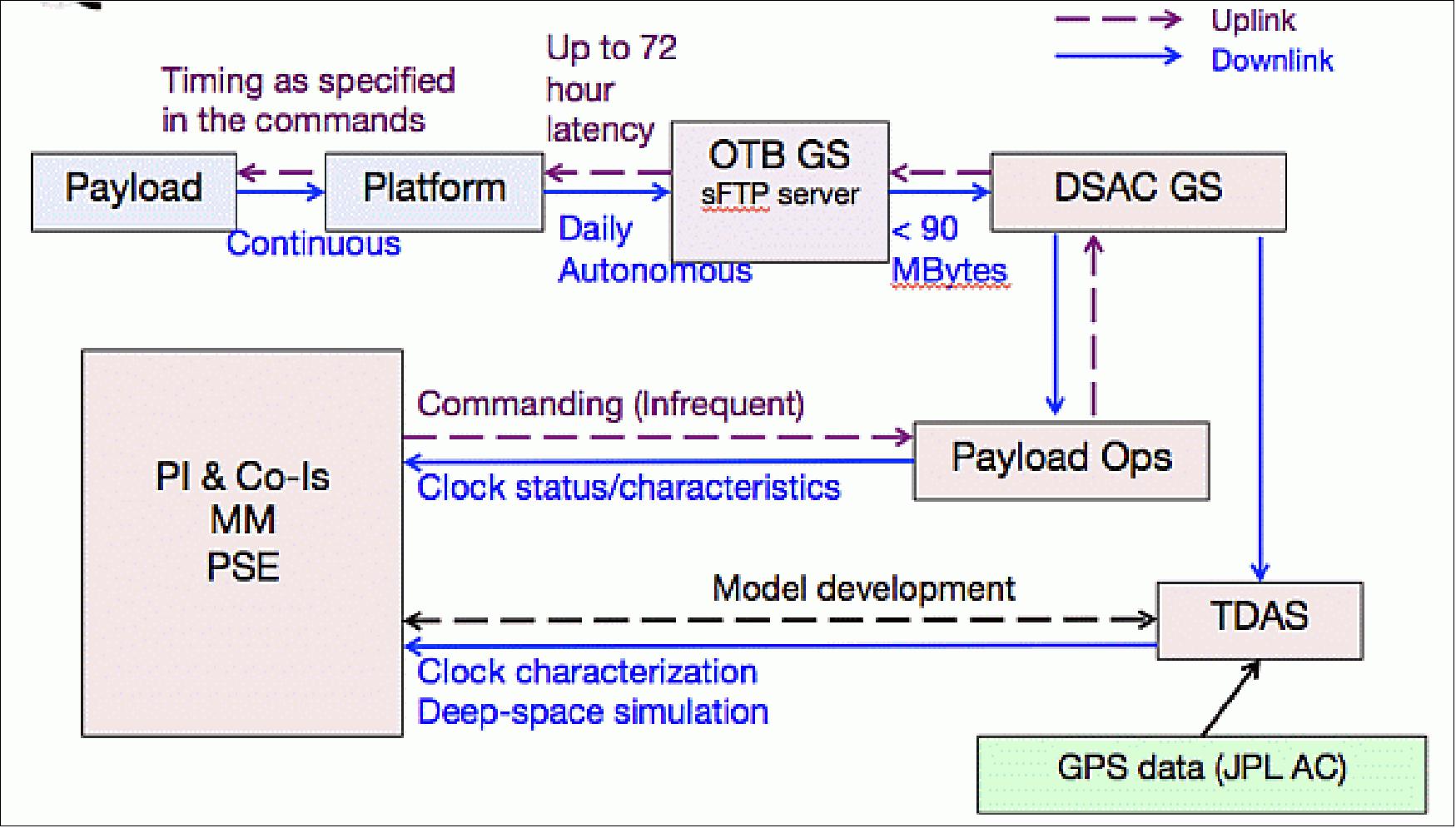
Key DSAC Performance Requirements
The overall DSAC mission needs to determine estimated clock stability (Allan Deviation or AD) of better than 2 x 10-14 at one day assuming that the clock itself is performing at or better than 1 x 10-14 at one day. This can be decomposed into several separate parts:
1) DSAC demonstrates a ground-based stability of better than 3 x 10-15 at one-day in an adiabatic laboratory environment when compared with a hydrogen maser.
2) DSAC demonstrates a spaceborne stability of better than 1 x 10-14 at one-day, where margin has been added to the prior requirement because a changing spacecraft environment (i.e., variable temperature, magnetic fields, radiation) affects clock stability.
3) Verification of DSAC’s spaceborne performance introduces an error of no-more than 5 x 10-15 AD at one-day (assuming a perfect input clock) in the overall clock estimation process.
Even though the key requirements specify stability at one day, the project will investigate clock performance on a large range of time scales. On shorter time scales, below 10 seconds, the stability of the USO driving DSAC is the key performance factor. In the 1 – 10 second range, the USO has a stability of better than 2 x 10-13. Fundamentally, the clock control process improves on this stability level over longer time scales and asymptotically approaches a white frequency noise characteristic until it reaches (typically on the order of days) some ‘floor’ level performance. Another objective of the DSAC mission is to observe and determine this performance ‘floor’.
If DSAC is able to perform at its prescribed levels, then its utility for one-way radiometric deep-space navigation can be validated by showing that orbit determination performance using the one-way data performs as well as or better than its traditional two-way counterpart. To this end, another key mission requirement is to demonstrate orbit determination uncertainty, in a scenario that represents typical deep-space navigation conditions, of less than 10 m (3σ) using one-way radiometric tracking data with measurement quality, quantity, and schedule characteristics (such as track duration and data gaps) that are operationally similar to that available in deep space navigation.
DSAC Theory
How an atomic clock will get humans to Mars on time
NASA navigators are helping build a future where spacecraft could safely and autonomously fly themselves to destinations like the Moon and Mars.
Navigators today tell a spacecraft where to go by calculating its position from Earth and sending the location data to space in a two-way relay system that can take anywhere from minutes to hours to deliver directions. This method of navigation means that no matter how far a mission travels through the solar system, our spacecraft are still tethered to the ground, waiting for commands from our planet.
That limitation poses obvious problems for a future crewed mission to another planet. How can astronauts navigate far from Earth if they don't have immediate control over where they're going? And how can they accurately land on another planet when there's a communication delay that affects how quickly they can adjust their trajectory into the atmosphere?
NASA's Deep Space Atomic Clock is a toaster-size device that aims to answer those questions. It's the first GPS-like instrument small and stable enough to fly on a spacecraft. The technology demonstration enables the spacecraft to know where it is without needing to rely on that data from Earth. In late June, the clock will launch on the SpaceX Falcon Heavy rocket into Earth's orbit for one year, where it will test whether it can help spacecraft locate themselves in space.
If the Deep Space Atomic Clock's trial year in space goes well, it could pave the way for a future of one-way navigation in which astronauts are guided by a GPS-like system across the surface of the Moon or can safely fly their own missions to Mars and beyond.
"Every spacecraft exploring deep space is steered by navigators here on Earth. Deep Space Atomic Clock will change that by enabling onboard autonomous navigation, or self-driving spacecraft," said Jill Seubert, the deputy principal investigator.
There's No GPS in Deep Space
Atomic clocks in space aren't new. Every GPS device and smartphone determines its location via atomic clocks on satellites orbiting Earth. The satellites send signals from space, and the receiver triangulates your position by measuring how long the signals take to reach your GPS.
Currently, spacecraft flying beyond Earth's orbit don't have a GPS to find their way through space. Atomic clocks on GPS satellites aren't accurate enough to send directions to spacecraft, when being off by even less than a second could mean missing a planet by miles.
Instead, navigators use giant antennas on Earth to send a signal to the spacecraft, which bounces it back to Earth. Extremely precise clocks on the ground measure how long it takes the signal to make this two-way journey. The amount of time tells them how far away the spacecraft is and how fast it's going. Only then can navigators send directions to the spacecraft, telling it where to go.
"It's the same exact concept as an echo," said Seubert. "If I'm standing in front of a mountain and I shout, the longer it takes for the echo to come back to me, the farther away the mountain is."
Two-way navigation means that no matter how deep into space a mission goes, it still has to wait for a signal carrying commands to cross the vast distances between planets. It's a process made famous by Mars landings like Curiosity, when the world waited 14 long minutes with mission control for the rover to send the message that it landed safely. That delay is an average wait time: Depending on where Earth and Mars are in their orbits, it can take anywhere from 4 to 20 minutes for a one-way signal to travel between planets.
It's a slow, laborious way to navigate in deep space, one that ties up the giant antennas of NASA's Deep Space Network like a busy phone line. During this exchange, a spacecraft flying at tens of thousands of miles per hour could be in an entirely different place by the time it "knows" where it is.
A Better Way to Navigate
An atomic clock small enough to fly on a mission but precise enough to give accurate directions could eliminate the need for this two-way system. Future navigators would send a signal from Earth to a spacecraft. Like its Earthly cousins, the Deep Space Atomic Clock onboard would measure the amount of time it took that signal to reach it. The spacecraft could then calculate its own position and trajectory, essentially giving itself directions.
"Having a clock onboard would enable onboard radio navigation and, when combined with optical navigation, make for a more accurate and safe way for astronauts to be able to navigate themselves," said Deep Space Atomic Clock Principal Investigator Todd Ely.
This one-way navigation has applications for Mars and beyond. DSN antennas would be able to communicate with multiple missions at a time by broadcasting one signal into space. The new technology could improve the accuracy of GPS on Earth. And multiple spacecraft with Deep Space Atomic Clocks could orbit Mars, creating a GPS-like network that would give directions to robots and humans on the surface.
"The Deep Space Atomic Clock will have the ability to aid in navigation, not just locally but in other planets as well. One way to think of it is as if we had GPS at other planets," said Eric Burt, the ion clock development lead.
Burt and fellow JPL clock physicists Robert Tjoelker and John Prestage created a mercury ion clock, which maintains its stability in space in the same way as refrigerator-size atomic clocks on Earth. In lab tests, the Deep Space Atomic Clock proved to be 50 times more accurate than GPS clocks. That's an error of 1 second every 10 million years.
The clock's demonstration in space will determine whether it can remain stable in orbit. If it does, a Deep Space Atomic Clock could fly on a mission as early as the 2030s. The first step toward self-driving spacecraft that could one day carry humans to other worlds.
The Deep Space Atomic Clock is hosted on a spacecraft provided by General Atomics Electromagnetic Systems of Englewood, Colorado. It is sponsored by the Technology Demonstration Missions program within NASA's Space Technology Mission Directorate and the Space Communications and Navigations program within NASA's Human Exploration and Operations Mission Directorate. JPL manages the project.
TDAS (Technology Demonstration Analysis System)
The primary software tools that make up TDAS include GIPSY-OASIS (GNSS-Inferred Positioning System and Orbit Analysis Simulation) and MONTE (Mission-analysis, Operations, and Navigation Toolkit Environment). Each of these are separate NASA-funded capabilities that represent the state-of-the art for Earth orbit determination using GPS data (GIPSY-OASIS) and deep space navigation (MONTE). The DSAC project is leveraging these tools for developing a precision clock determination capability that enables the project to meet its requirements. Given the a priori capabilities of these tools, the DSAC project need only develop specific capabilities needed for PCD and one-way deep space navigation. These enhancements are done in the context of the GIPSY-OASIS and MONTE projects. That is, they are funded by DSAC, but implemented and maintained by their respective development teams. The benefit is the new capabilities receive full operational software pedigrees, and, in addition to DSAC, are available to any other mission that might need them now or in the future. Specific to MONTE, development of precision one-way radiometric measurement modeling, high fidelity clock modeling, and appropriate filter methods is a key feed-forward infusion capability to future missions that would fly DSAC.
The GIPSY-OASIS software is currently used for POD (Precise Orbit Determination) of LEO spacecraft that carry GPS receivers such as gravity missions (e.g., CHAMP and GRACE) and altimetry missions (e.g., TOPEX/Poseidon, Jason-1, Jason-2/OSTM) as well by the geodetic community to determine the precise location of hundreds of ground GPS receivers. It is also used by the JPL IGS Analysis Center to determine the GPS constellation orbits and clocks. These orbit and clock solutions are then combined with additional, independent GPS clock and orbit solutions at the IGS Analysis Coordination Center to produce the IGS GPS orbit and clock solution. Several features of GIPSY-OASIS make it the software of choice to study the performance of the DSAC clock in space, among them:
• Unlike other POD software packages, GIPSY-OASIS does not eliminate the receiver clock error using differenced observables. It is this receiver clock error x (t) that is used to determine the DSAC clock stability.
• GIPSY-OASIS has been used extensively in operations and is continuously upgraded to remain at the state of the art. The DSAC mission will benefit from many of these upgrades.
The MONTE software has been and continues to be developed to serve as the next generation software of choice for deep space navigation and mission design. MONTE is a highly-capable and flexible software tool for trajectory integration, trajectory targeting, orbit determination, and statistical maneuver analysis. MONTE, previously known as the Next Generation Navigation Software, development started in the late 1990’s with a charter to upgrade and replace the Fortran 77-based Double Precision Orbit Determination Program (DPTRAJ/ODP) developed in the mid-1960s. MONTE’s core computational capabilities are written in C++ and it has a Python-based script/interactive shell user interface. Initially released in 2000 and adopted for operational use in 2006 (by the Mars Phoenix mission), MONTE is currently utilized by JPL flight projects and is continually evolving to include mission- and user-requested enhancements. MONTE has also been released for university research. Though primarily created for deep space navigation and mission design, MONTE is increasingly supporting GPS-tracking Earth orbiters such as GRACE Follow-On and DSAC.
The GIPSY-OASIS software package has been the primary tool for simulating DSAC’s expected PCD performance, while MONTE has been used as an independent check of these results. The nominal mission performance results presented in the sequel have been generated by GIPSY-OASIS, and are being used by the project during payload development to ensure DSAC’s stability requirements (with associated AD specifications) will be met during operations. MONTE’s primary role has been to determine that one-way data derived from DSAC and associated filter designs are sufficient to meet the analog deep space navigation performance requirement (i.e., 10 m (3σ) using a deep space tracking schedule and orbit determination filter design).
ETB (Electronics Test Bed)
The Electronic Test Bed seeks to evaluate and demonstrate a number of new electronic components, processors, and memory devices, enabling in-orbit heritage to be gained on components that may be incorporated into future designs. 35)
• FLexRX (Flexible Receiver): The incorporation of FlexRX, Surrey’s next-generation programmable receiver equipment, allows in-orbit test, demonstration, and qualification of a product that will be used on future missions and also sold to third parties. 36)
FlexRX is an enhanced version of a legacy device flown on numerous earlier missions. A FlexRX twin is already in orbit and being tested on the Surrey TechDemoSat-1 launched in July 2014. The performance data collected during the OTB-1 and TechDemoSat-1 missions will help to quickly validate the receiver’s readiness for our own operational use and future use by our customers.
The most important technological advancement in FlexRX is its ability to receive data transmitted from the ground at higher uplink rates than the receivers used now. The telemetry commands are received from controllers on the ground, transmitted in the form of radio signals from ground stations. These commands program the satellite to perform various tasks and maintain its overall operation, keeping it safely in orbit.
Satellites can only receive the telecommand data when they have line-of-sight contact with the station. Depending on the orbit of the satellite, this connection time may last just a few minutes before the satellite passes out of sight over the horizon. If the time is brief, the ground station may not be able to transmit all of the necessary commands to the satellite. An obvious solution to this problem is to transmit and receive telemetry at a faster rate.
Surrey has designed the FlexRX to handle incoming data transmissions at a variety of rates. Once we launch OTB-1 in 2016, FlexRX will serve as the primary instrument receiving ground station commands. The goal will be to transmit information from the ground at different rates to confirm the highest stable uplink rate possible for the new receiver throughout the ground station passes. Satellite performance data beamed back to the ground station through a separate instrument will tell us the maximum rate achieved.
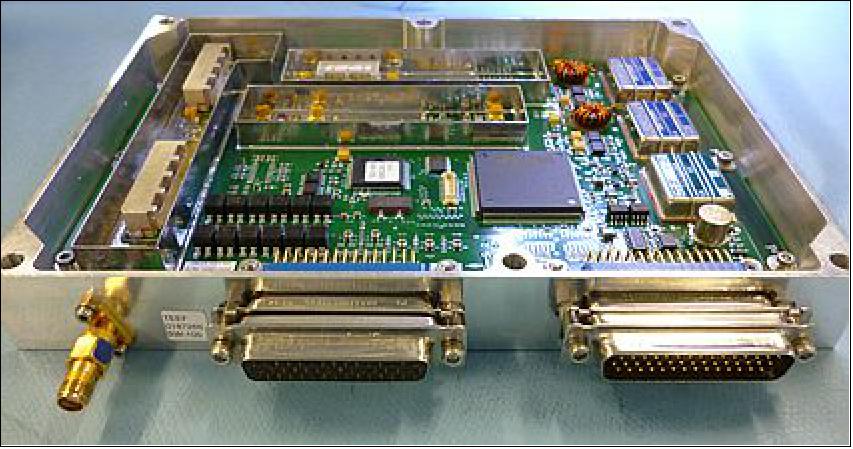
• RadMon (Radiation effects Monitor): The latest generation RadMon device will collect data on the radiation environment in space for correlation with other OTB sensors and for applications in future Surrey missions. RadMon will continuously collect radiation data, providing information on the doses to which OTB components are exposed during the mission. Research by NASA has concluded that electronic microchips begin to suffer performance errors with their memory storage in the 15 to 20 krad TID (total ionizing dose) range. These errors can wreak havoc on the function of a satellite or its subsystems. 37)
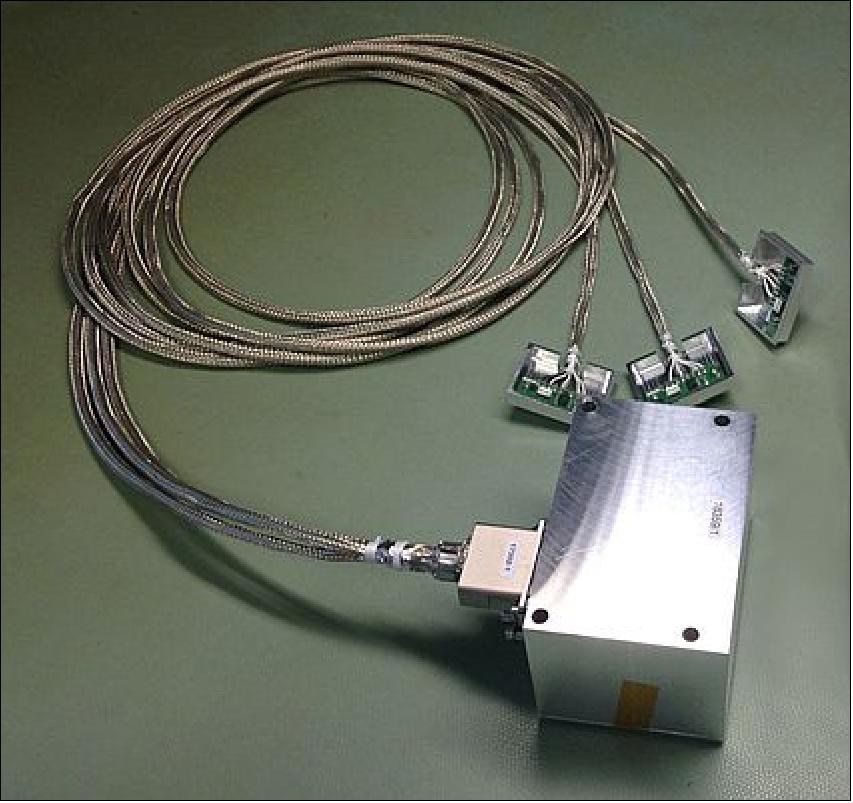
The RadMon will perform three functions—total dose measurement, particle detection, and dose-rate detection. Dose-rate detection will pick up high and low areas of radiation, helping to spot those regions that have high levels of radiation, such as the SAA (South Atlantic Anomaly). The RadMon payload will allow the project to plot this anomaly.
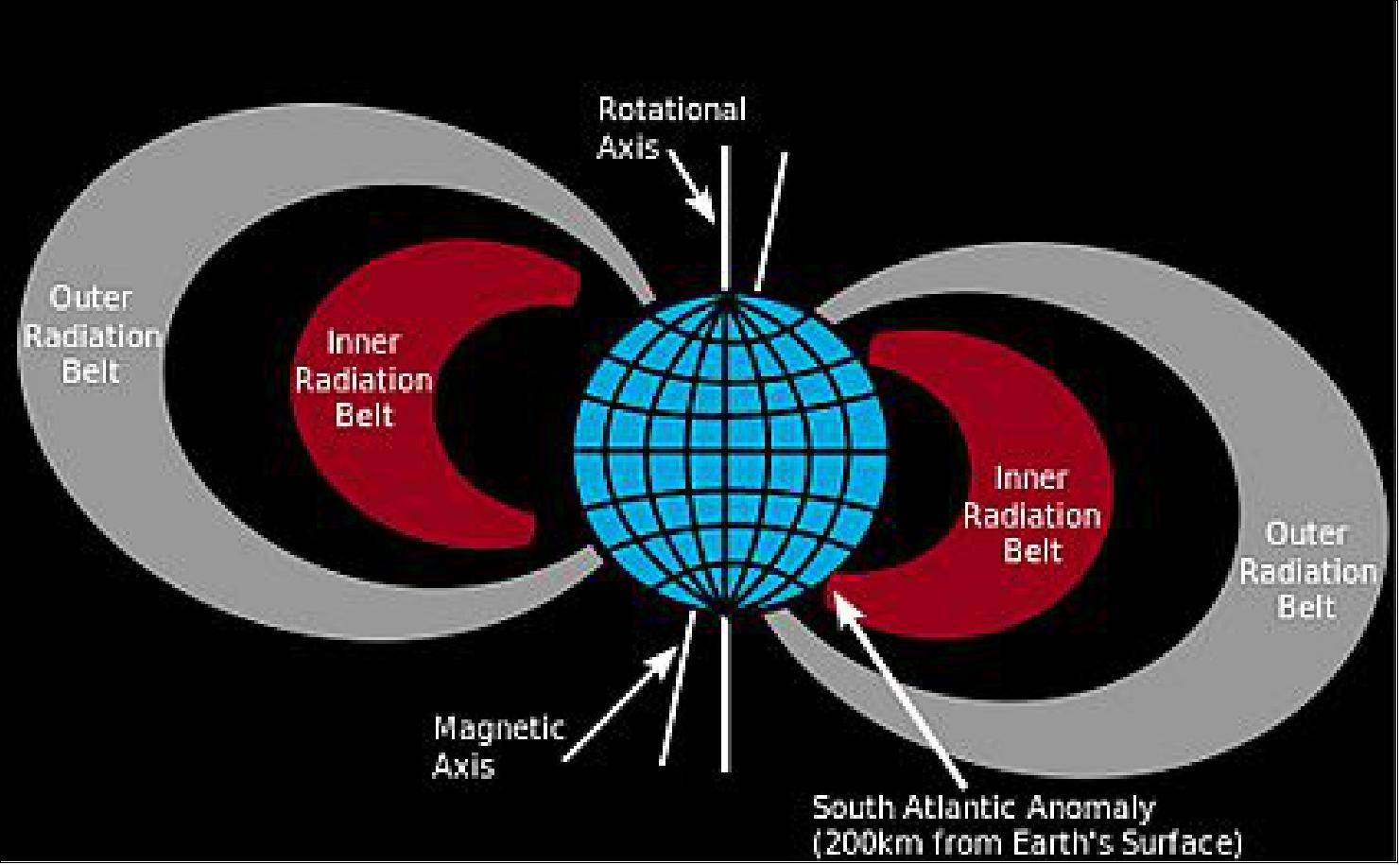
MSA (Modular Solar Array)
Developed by Vanguard Space Technologies for the U.S. AFRL (Air Force Research Laboratory) in Albuquerque, NM, the MSA will demonstrate flight readiness of a standardized modular approach to solar panels, with the ability for modules to be quickly replaced during final satellite testing prior to launch. Space-qualifying a system with this degree of interchangeability and change-out capability has the potential to reduce schedule delays (and therefore cost) before launch, when there is typically very little remaining schedule margin.
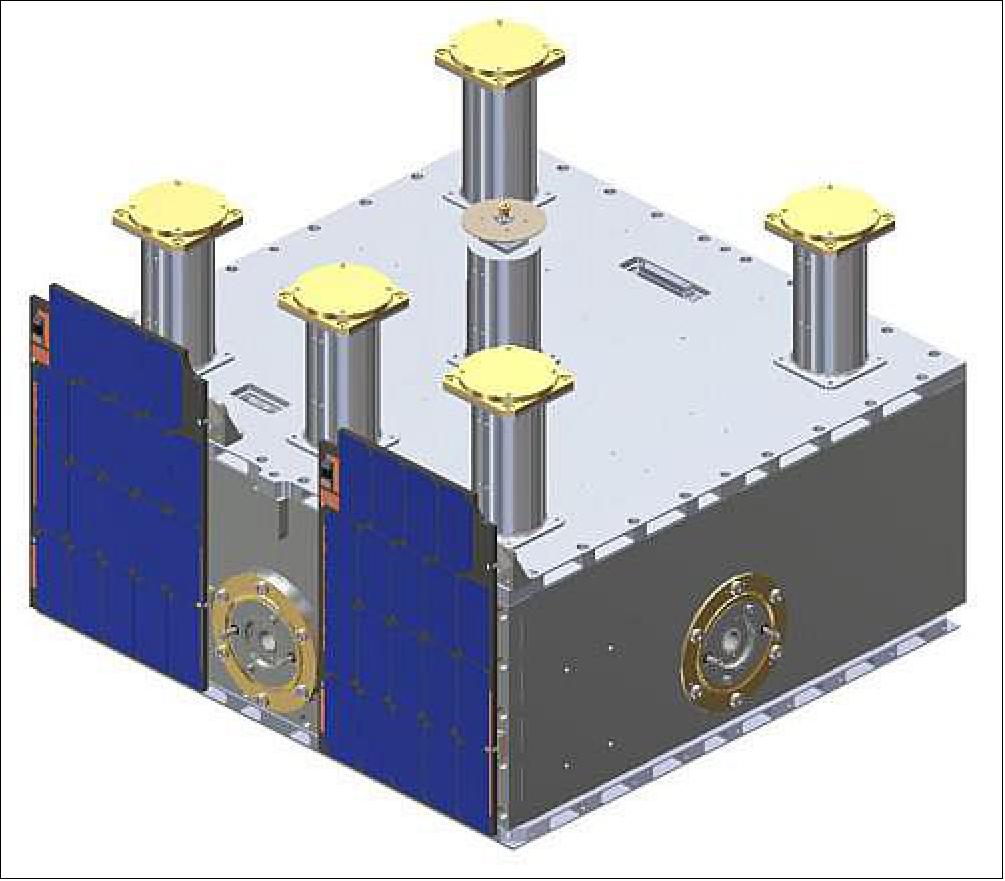
iMESA-R (Integrated Miniaturized Electrostatic Analyzer-Reflight)
Developed by cadets at the USAFA (U.S. Air Force Academy) in Colorado Springs, iMESA-R is a miniaturized sensor that will sample the space environment to find plasma irregularities that may forecast outages in space weather models. While iMESA instruments have flown on previous USAF missions, the variant flying on OTB-1 features a new miniaturized dosimeter design.
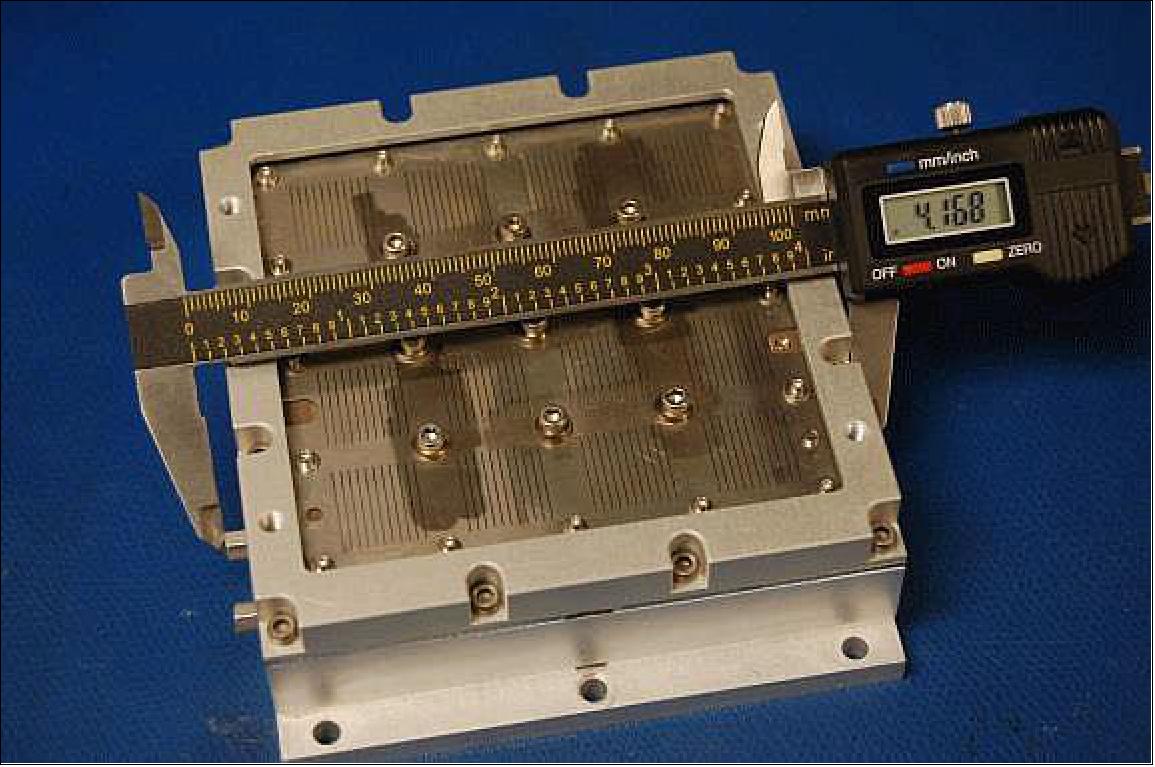
CUSP (Colorado University Surrey Project)
A joint project between Surrey and the University of Colorado, Boulder, CUSP is a data collection and storage electronic test bed experiment built by students using off-the-shelf components.
In addition to carrying the above five payloads, OTB will test a Terminator Tape Deorbit Module—a device developed by TUI (Tethers Unlimited Inc.) of Bothell, Washington, USA, to deorbit the OTB satellite at the end of its planned mission. TUI used their Cubesat tether design as the basis for this new, larger Small Satellite tether. The OTB mission will be the first Surrey spacecraft to fly a tether-based deorbit system. This passive de-orbit device is an ideal mass, power, volume, and cost-efficient solution to complement the business case closing objectives of the OTB mission; any typical fluid or solid-based propulsion device would impact the payload carrying capabilities of the satellite platform, in addition to requiring active control at end of life and additional redundancy and safety mechanisms.
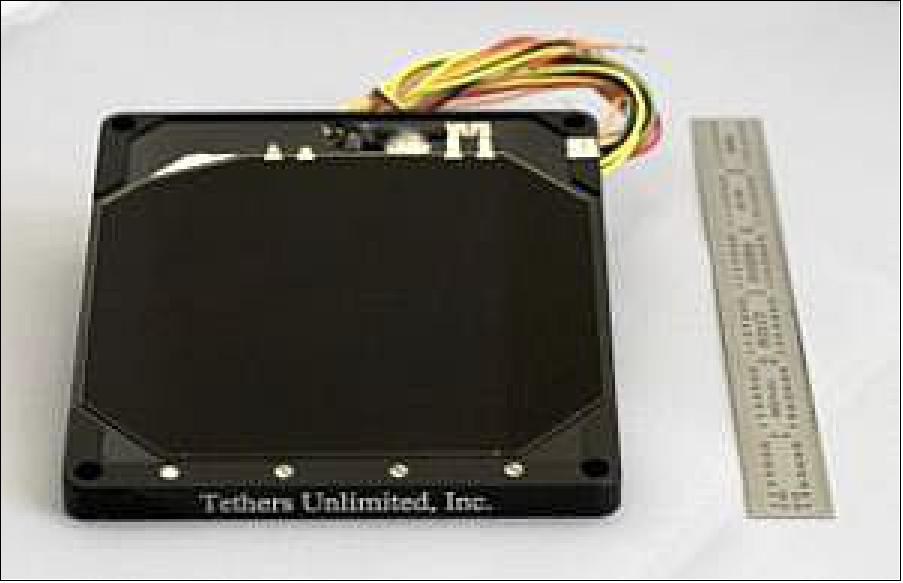
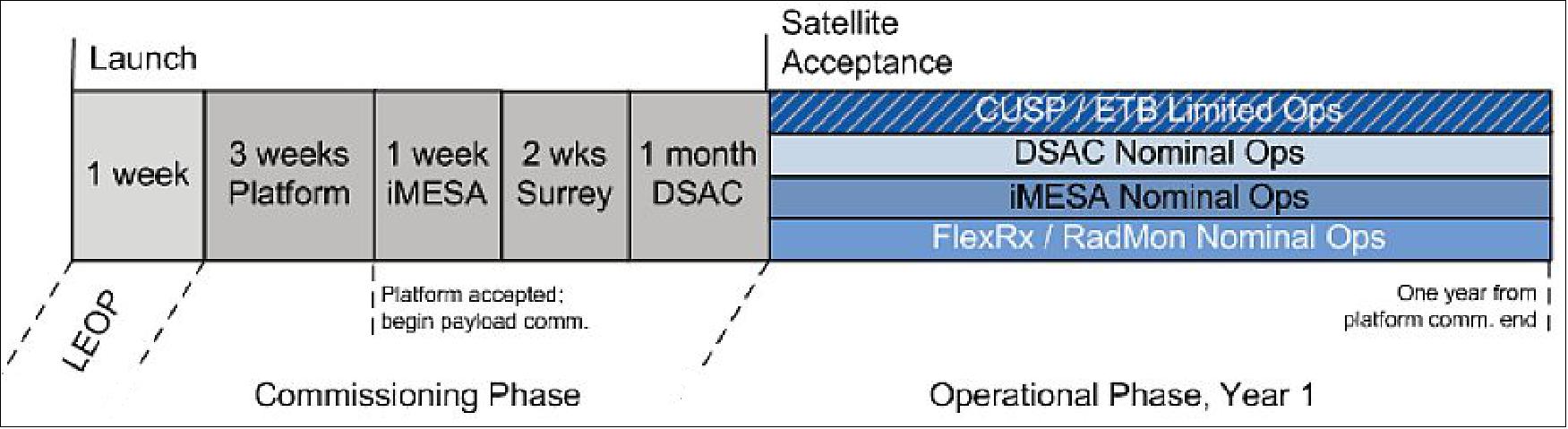
References
1) ”OTB: The Mission,” SST-US, URL: https://web.archive.org/web/20161212232954/http://www.sst-us.com/missions/otb/otb/otb-the-mission
2) Danny Baird, ”NASA Tests Atomic Clock for Deep Space Navigation,” NASA, 6 Feb. 2018, URL: https://www.nasa.gov/feature/goddard/2018/nasa-tests-atomic-clock-for-deep-space-navigation
3) ”NASA Selects Surrey Satellite US for Flight of Deep Space Atomic Clock Payload,” SST-US, June 26, 2013, URL: https://web.archive.org/web/20170121002603/http://www.sst-us.com/press-1/nasa-selects-surrey-satellite-us-for-flight-of-dee
4) ”Surrey Satellite US Announces US Air Force Hosted Payload Contract Award,” July 16, 2014, URL: https://web.archive.org/web/20141226173720/http://www.sst-us.com/news-and-events/2014-news-archive/pr_2014_07_smc_hops
5) F. Brent Abbott, William Thompson, Todd A. Ely, ”Hosting the Deep Space Atomic Clock (DSAC) on the Orbital Test Bed (OTB-1) satellite,” 29th annual AAS Guidance and Control Conference, Breckenridge, CO, USA, Feb. 1-5, 2014, paper: AAS 14-075
6) Anita Bernie, Tyler Murphy, ”The Technology Demonstration Objectives of the Orbital Test Bed Mission: Using the Hosted Payload Concept to Advance Small Satellite Technologies and Scientific Capabilities,” Proceedings of the 28th Annual AIAA/USU Conference on Small Satellites, Logan, Utah, USA, August 2-7, 2014, paper: SSC14-VII-8, URL: http://digitalcommons.usu.edu
/cgi/viewcontent.cgi?article=3065&context=smallsat
7) Brent Abbott, ”Changing the Economics of Space — Surrey Satellite Technology US LLC,” June 2015, URL: https://web.archive.org/web/20190423034300/https://www.sprsa.org/sites/default/files/conference-presentation/2015_06_PayloadHostingBusiness%20Final.pdf
8) ”General Atomics Awarded NASA Contract for Commercial Satellite,” GA-EMS, 17 September 2018, URL: http://www.ga.com/general-atomics-awarded-nasa-contract-for-commercial-satellite
9) ”General Atomics Completes Ready-For-Launch Testing of Orbital Test Bed Satellite,” GA-EMS, 23 April 2018, URL: http://www.ga.com/
general-atomics-completes-ready-for-launch-testing-of-orbital-test-bed-satellite
10) ”General Atomics Acquires Assets of Surrey-US, LLC,” General Atomics, 13 November 2018, URL: http://www.ga.com/general-atomics-acquires-assets-of-surrey-us-llc
11) Stephen Clark, ”Falcon Heavy launches on military-led rideshare mission, boat catches fairing,” Spaceflight Now, 25 June 2019, URL: https://spaceflightnow.com/2019/06/25/
falcon-heavy-launches-on-military-led-rideshare-mission-boat-catches-fairing/
12) “GPIM Spacecraft to Validate Use of 'Green' Propellant,” NASA, Aug. 19, 2014, URL: http://www.nasa.gov/content/gpim-spacecraft-to-validate-use-of-green-propellant/
13) ”General Atomics Orbital Test Bed Satellite Successfully Launched and Deployed,” GA-EMS, 25 June 2019, URL: http://www.ga.com/
general-atomics-orbital-test-bed-satellite-successfully-launched-on-board-spacex-falcon-heavy
14) ”NASA Technology Missions Launch on SpaceX Falcon Heavy,” NASA Release 19-049, 25 June 2019, URL: https://www.nasa.gov/press-release/nasa-technology-missions-launch-on-spacex-falcon-heavy/
15) ”Working Overtime: NASA’s Deep Space Atomic Clock Completes Mission,” NASA/JPL, 05 October 2021, URL: https://www.jpl.nasa.gov/news/
working-overtime-nasas-deep-space-atomic-clock-completes-mission?utm_source=iContact&
utm_medium=email&utm_campaign=nasajpl&utm_content=daily20211005-1
16) ”Deep Space Atomic Clock Moves Toward Increased Spacecraft Autonomy,” NASA/JPL, 30 June 2021, URL: https://www.jpl.nasa.gov/news/
deep-space-atomic-clock-moves-toward-increased-spacecraft-autonomy?utm_source=iContact&
utm_medium=email&utm_campaign=nasajpl&utm_content=daily20210630-3
17) E. A. Burt, J. D. Prestage, R. L. Tjoelker, D. G. Enzer, D. Kuang, D. W. Murphy, D. E. Robison, J. M. Seubert, R. T. Wang & T. A. Ely, ”Demonstration of a trapped-ion atomic clock in space,” Nature, Volume 595, pp: 43-47, Published: 30 June 2021, https://doi.org/10.1038/s41586-021-03571-7
18) Calla Cofield, ”NASA Extends Deep Space Atomic Clock Mission,” NASA/JPL News, 24 June 2020, URL: https://www.jpl.nasa.gov/news/news.php?feature=7687
19) ”What Is an Atomic Clock? And the Deep Space Atomic Clock?, ” SciTechDaily, 11 May 2020, URL: https://scitechdaily.com/what-is-an-atomic-clock-and-the-deep-space-atomic-clock/
20) ”General Atomics Announces NASA Deep Space Atomic Clock in Nominal Operation,” GA-EMS, 20 December 2019, URL: https://www.ga.com/
general-atomics-announces-nasa-deep-space-atomic-clock-in-in-nominal-operation
21) ”NASA Activates Deep Space Atomic Clock,” NASA News, 26 August 2019, URL: https://www.nasa.gov/feature/jpl/nasa-activates-deep-space-atomic-clock
22) ”Viasat's Real-Time Earth Ground Service Provided Commissioning and Operations Support to General Atomics Electromagnetic Systems' Orbital Test Bed Satellite,” PRnewswire, 23 July 2019, URL: https://www.prnewswire.com/news-releases/viasats-real-time-earth-ground-service-provided-commissioning-and-operations-support-to-general-atomics-electromagnetic-systems-orbital-test-bed-satellite-300889228.html
23) ”Five Payloads Sharing the Ride on Upcoming OTB Mission,” SST-US, 2014, URL: https://web.archive.org/web/20180720222753/http://www.sst-us.com/blog/july-2014/five-payloads-sharing-the-ride-on-upcoming-otb-mis
24) “NASA Selects Surrey Satellite US for Flight of Deep Space Atomic Clock Payload,” SSTL, June 27, 2013, URL: https://web.archive.org/web/20160408070300/http://www.sstl.co.uk/Press/NASA-Selects-Surrey-Satellite-US-for-Flight-of-Dee
25) “Deep Space Atomic Clock (DSAC),” NASA, Dec. 2011, URL: http://www.nasa.gov/mission_pages/tdm/clock/clock_overview.html
26) “NASA to Fly Deep Space Atomic Clock to Improve Navigation Technology,” NASA, April 9. 2012, URL: http://www.nasa.gov/mission_pages/tdm/clock/dsac.html
27) “Deep Space Atomic Clock,” NASA Fact Sheet, URL: http://www.nasa.gov/sites/default/files/files/DSAC_Fact_Sheet.pdf
28) John D. Prestage, “Next Generation Space Atomic Clock, Space Communications and Navigation (SCaN) Technology,” NASA/JPL, 2011, URL: http://scpnt.stanford.edu/pnt/PNT11/2011_presentation_files/19_Prestage-PNT2011.pdf
29) “Deep Space Atomic Clock (DSAC),” NASA, Oct. 9, 2012, URL: http://www.nasa.gov/directorates/heo/scan/engineering/technology/txt_dsac.html#.U5G91Xa4T5o
30) Todd A. Ely, John Prestage, Robert Tjoelker, Timothy Koch, Da Kuang, Karen Lee, David Murphy, Jill Seubert, “The Deep Space Atomic Clock Mission,” Proceedings of the 23rd International Symposium on Space Flight Dynamics, Pasadena, CA, USA, Oct. 29- Nov. 2, 2012, URL: http://issfd.org/ISSFD_2012/ISSFD23_OD1_2.pdf
31) Todd A. Ely, Jill Seubert, Julia Bell, “Advancing Navigation, Timing, and Science with the Deep Space Atomic Clock,” SpaceOps 2014, 13th International Conference on Space Operations, Pasadena, CA, USA, May 5-9, 2014, paper: AIAA 2014-1856, URL: http://arc.aiaa.org/doi/pdf/10.2514/6.2014-1856
32) Todd A. Ely, David Murphy, Jill Seubert, Julia Bell, Da Kuang, “Expected performance of the Deep Space Atomic Clock mission,” Proceedings of the 24th AAS/AIAA Space Flight Mechanics Meeting, Santa Fe, NM, USA, January 26 –30, 2014, paper: AAS 14-254
33) Todd A. Ely, Jill Seubert, ”One-way radiometric navigation with the Deep Space Atomic Clock,” 25th AAS/AIAA Space Flight Mechanics Meeting, Williamsburg, VA, USA, January 11-15, 2015, paper: AAS 15-384
34) ”How an Atomic Clock Will Get Humans to Mars on Time,” NASA/JPL News, 14 June 2019, URL: https://www.jpl.nasa.gov/news/news.php?release=2019-116
35) ”OTB Payload Profile: RadMon to Measure Radiation in Low-Earth Orbit,” SST-US, 2014, URL: https://web.archive.org/web/20141227134805/http://www.sst-us.com/blog/october-2014/otb-payload-profile-radmon-to-measure-radiation-in
36) ”OTB Blog Series: FlexRX Receiver to Gain In-Orbit Heritage,” SST-US, 2015, URL: https://web.archive.org/web/20150710235231/http://www.sst-us.com/blog/may-2015/otb-payload-profile-flexrx-receiver-to-gain-in-orb
37) ”OTB Payload Profile: RadMon to Measure Radiation in Low-Earth Orbit,” SST-US, 2014, URL: https://web.archive.org/web/20141227134805/http://www.sst-us.com/blog/october-2014/otb-payload-profile-radmon-to-measure-radiation-in
The information compiled and edited in this article was provided by Herbert J. Kramer from his documentation of: ”Observation of the Earth and Its Environment: Survey of Missions and Sensors” (Springer Verlag) as well as many other sources after the publication of the 4th edition in 2002. - Comments and corrections to this article are always welcome for further updates (eoportal@symbios.space).
Spacecraft Launch Mission Status Sensor Complement References Back to Top