ISARA (Integrated Solar Array and Reflectarray Antenna)
Non-EO
NASA-JPL
Quick facts
Overview
Mission type | Non-EO |
Agency | NASA-JPL |
Launch date | 12 Nov 2017 |
ISARA (Integrated Solar Array and Reflectarray Antenna)
Spacecraft Launch Mission Status Experiment Complement References
ISARA is a NASA/JPL (Jet Propulsion Laboratory) nanosatellite (3U CubeSat) demonstration mission with the goal to demonstrate a Ka-band reflectarray antenna that will increase the downlink data rates for small spacecraft from the typical existing rates from a baseline of 9.6 kbit/s to over 100 Mbit/s, a hundredfold increase in data capacity. For a modest increase in mass, volume, and cost, the high data rate this technology enables will pave the way for high value science missions and formation flying missions that utilize distributed CubeSats and small satellites. 1)
ISARA is a JPL PM-led mission that will be carried out in close collaboration with Pumpkin, Inc. and Aerospace Corp. In February 2013, ISARA was down-selected for a launch opportunity through the NASA ELaNa program.
The ISARA mission is funded through NASA's SSTP (Small Spacecraft Technology Program), a program within the new Space Technology Mission Directorate which was formed as a catalyst for the creation of technologies and innovation needed to maintain NASA leadership in space, while also benefiting America's economy. The SSTP was created specifically to develop and demonstrate new technologies and capabilities for small spacecraft.
ISARA Rideshare Opportunity
In 2014, The Aerospace Corporation proposed an idea for a secondary payload to fill approximately 1U of unused volume on the ISARA spacecraft. The primary ISARA mission required a 3U bus to accommodate the reflectarray antennae/solar panel structure, but used less than 2U of spacecraft volume. To fill the remaining volume, a combination of two readily available compact uncooled IR cameras was chosen, and a visible camera similar to those flown on prior AeroCube missions, but with greater low-light sensitivity. The Space and Missile System Center's Advanced Development program office (SMC/AD) was interested in gaining experience with flying COTS camera hardware and funded the secondary payload effort. 2)
The sensor capabilities matched specific nighttime remote sensing science goals of The Aerospace Corporation's Space Science Applications Laboratory, including exploring the utility of the near infrared for nighttime cloud detection and combining cloud and nightlight sensors on a single payload. 3)
Payload development was greatly speeded by use of the 5-camera controller card technology for the OCSD/AC-7 satellite. Some added software was necessary for this card to control the new cameras. Power conditioning boards were also necessary for the IR cameras. As mentioned above, two camera cards were necessary, as the main payload had two star sensors, a nadir-pointed fisheye camera, and a reflectarray deployment fisheye camera. Redundant boards also ensured there was less risk to the main reflectarray experiment. The new imaging instruments were as isolated as possible from the primary experiment.
Spacecraft
ISARA is a 3U bus to host two payloads, namely the ISARA (Integrated Solar Array and ReflectArray) antenna as primary payload and the CUMULOS (CubeSat Multispectral Observation System), an experimental remote sensing payload of The Aerospace Corporation.
A nominal five month Space Flight Demonstration will be used to confirm a 100 Mbit/s data rate and verify antenna performance to TRL 7 (Technology Readiness Level 7). The spacecraft is a 3U CubeSat carrying a Ka-band payload that includes a low power transmitter, HGA (High Gain Antenna), standard gain reference antenna and RF antenna select switch. A Ka-band ground station will verify high data rate by SNR (Signal-to-Noise) measurement and measure the antenna performance. The HGA gain will be measured by switching between the HGA and an on-board SGA (Standard Gain Antenna), while the spacecraft will be slewed on orbit to measure the antenna patterns. The on-orbit data will be compared to measurements that were taken prior to launch.
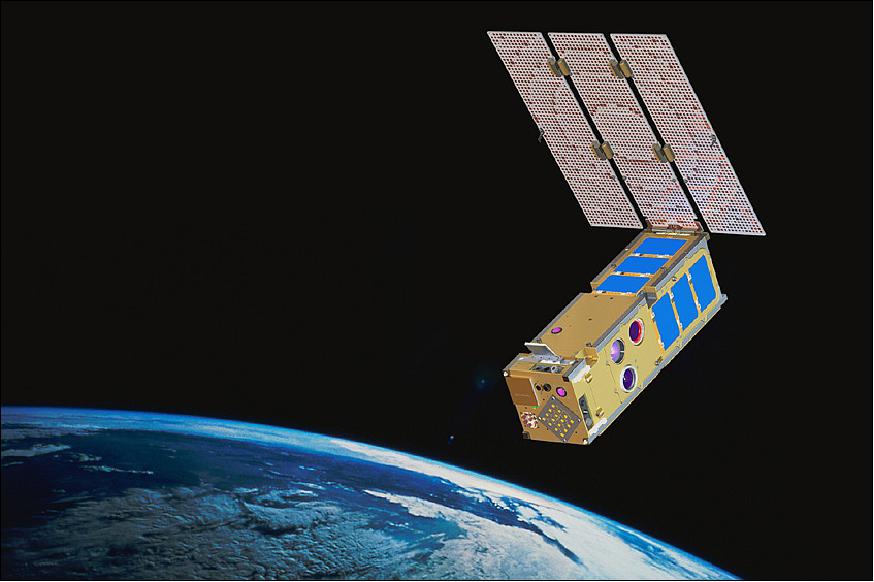
The reflectarray antenna consists of three panels, electrically tied together through hinges, which have an array of printed circuit board patches on them. The size of the patches are adjusted so that the phase of the reflected feed illumination collimates the radiation in much the same way a parabolic dish reflector would. Unlike a parabolic dish, however, the reflectarray panels are flat, which enables them to be folded down against the CubeSat. On the opposite side of the printed reflectarray antenna, solar cells have been added. This makes the overall antenna/solar array panel assembly slightly thicker, but the cells are stowed in the "dead space" between the launch rails that would have otherwise been left empty. This combination of antenna and solar cells makes for a very efficient use of CubeSat volume, leaving plenty of room for payloads such as science instruments or imaging systems. 4)
The ISARA technology will be validated in space during the mission to measure key reflectarray antenna characteristics, which include how much power can actually be obtained over its field of view. ISARA contains a transmitter and an avionics subsystem that features a GPS (Global Positioning System) receiver and a high precision attitude control system designed to orient the CubeSat to enable accurate antenna beam pointing. Once in orbit, ISARA will deploy its solar array and reflectarray antenna. It then will use its attitude determination and control system to stabilize itself. An ultra high frequency (UHF) communications system will be used to make initial contact with the satellite and perform in-orbit checkout procedures.
During the in-orbit test, ISARA's reflectarray antenna will transmit a signal that will be received by a ground station located at NASA/JPL ( Jet Propulsion Laboratory) in Pasadena, California. The spacecraft's location and orientation telemetry data will be analyzed to reconstruct the antenna signal pattern, which will then be compared against pre-flight ground measurements.
ISARA contains a transmitter and an avionics subsystem that features a high precision ADCS (Attitude Determination and Control Subsystem) along with a suite of ADCS sensors and actuators. A high accuracy MAI-400 ADCS is used to achieve the required 0.2° pointing accuracy. The standard bus avionics that are essentially identical to the OCSD (Optical Communication and Sensor Demonstration) 3U CubeSat,include a primary flight computer, two UHF radios and a GPS receiver for navigation. ISARA has a mass of ~5 kg.
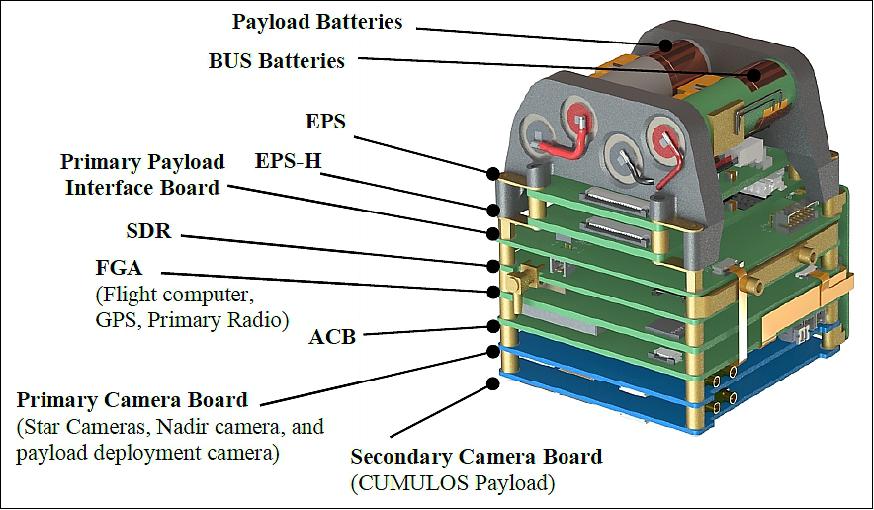
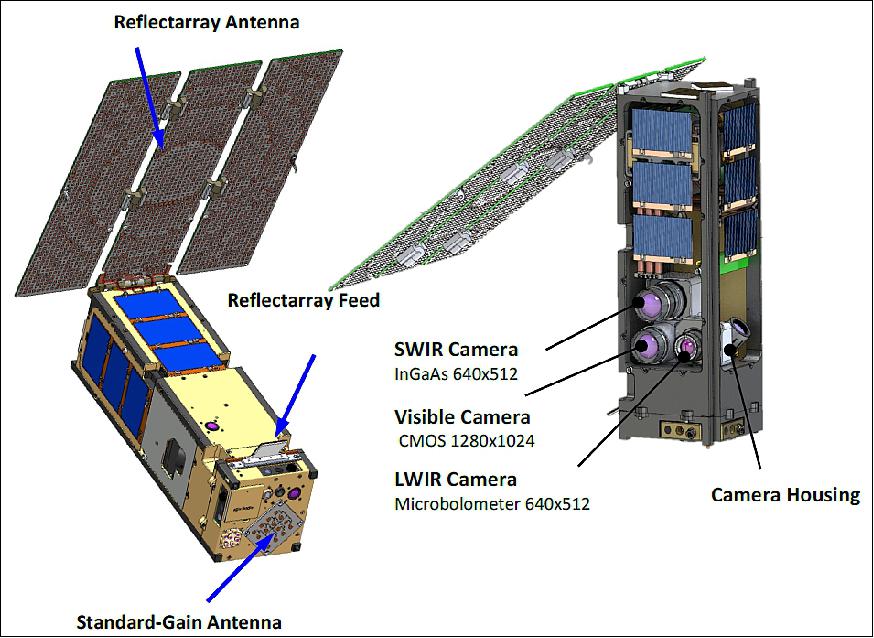
The stowage volume and spacecraft power provided by the ISARA technology also enabled the ISARA mission to carry a secondary payload known as CUMULOS (CubeSat Multispectral Observation System), an experimental Aerospace Corporation remote sensing payload. CUMULOS is composed of a 0.4-0.8 µm visible camera, a 0.9-1.7 µm short-wavelength infrared camera, and an 8.0-13.5 µm long-wavelength infrared, microbolometer camera.
The CUMULOS sensors provide a small-aperture, large field-of-view, remote sensing payload suitable for testing the performance of passively-cooled commercial sensors for weather and environmental monitoring missions. CUMULOS is designed for point-and-stare imaging and will allow almost simultaneous 3-band coverage of regions 230 x 180 km in size, at ground sample distances from 180 to 600 m from an orbital altitude of 600 km. Remote sensing applications to be investigated include: cloud cover detection, surface temperature measurement, hotspot detection (including fires, gas flares, and volcanic activity), and detection of nighttime lights.
At the end of the validation mission, the reflectarray antenna technology will be available for use on other missions that need high bandwidth telecommunications. The ISARA technology will enable CubeSats and other small satellites to serve as viable platforms for performing missions that were previously only possible on larger and more costly satellites. For a modest increase in mass, volume and cost, the high data rate this technology enables will pave the way for high value science missions and formation flying missions that use distributed CubeSats and small satellites.
KBE (Ka-Band Exciter): The KBE is a Ka-band (26 GHz) RF power amplifier designed specifically for ISARA. As the illustration in Figure 4 suggests, the KBE is a single circuit board that occupies 97mm x 97mm x 20mm of payload volume and wwith a mass of 200 gram. It is located adjacent to the Feed and the SGA in order to minimize transmission line losses. This amplifier produces 200 mW of RF power to meet the ISARA link budget requirements. This power amplifier is compatible with the recently developed IRIS software defined radio, 5) so a fully functional Ka-band telecom system could be developed in a short time.
A key component of the KBE is the RF single pole, double throw switch that toggles output power between the reflectarray and the SGA. As explained below, this is the mechanism used to calibrate gain measurements in the on-orbit test.
Launch
The ISARA mission was launched on November 12, 2017 on the Cygnus Orbital ATK CRS OA-8 Mission to the ISS on the upgraded Orbital ATK Antares 230 vehicle. The launch site was MARS (Mid-Atlantic Regional Spaceport) at NASA's Wallops Flight Facility in Virginia. 6) 7)
Orbit : Near-circular orbit, altitude of ~400 km to ISS, inclination =51.6°.
For the OA-8 mission, Orbital ATK is using the Enhanced Cygnus PCM (Pressurized Cargo Module) to deliver cargo to the space station. The cargo capability of the Enhanced Cygnus, developed by Thales Alenia Space, is more than 3500 kg with a total volumetric capacity of 27 m3 (the ascent cargo mass is 3,350 kg). The supply shipment includes:
- 1240 kg of crew supplies. Fresh fruit and vegetables are among the goodies riding inside a refrigerator on Cygnus spacecraft.
- 740 kg of science investigations
- 132 kg of spacewalk equipment
- 851 kg of vehicle hardware
- 34 kg of computer resources
The Orbital ATK CRS-8 (OA-8) launch carried another historic NanoRacks mission to the ISS. With a completely full ENRCSD (External NanoRacks Cygnus Deployer), a virtual reality camera, and educational research, this mission marks over 600 NanoRacks payloads delivered to the ISS since 2009. 8)
This mission is enabling a unique virtual reality opportunity with National Geographic's VUZE camera. Integrated and launched via NanoRacks, VUZE will allow for the recording of the new National Geographic series "One Strange Rock," in which the astronaut crew will record a series of virtual reality pieces for incorporation into a larger documentary about natural history and the solar system. This is National Geographic's first time launching with NanoRacks.
Additionally, OA-8 is the fourth mission in which NanoRacks is providing opportunities for CubeSat deployment from Cygnus after the vehicle departs from the station. The NanoRacks ENRCSD is installed on the exterior of the Cygnus service module with the capability to deploy satellites after Cygnus' completion of its primary ISS resupply mission.
On this ENRCSD mission, NanoRacks has 14 satellites ready to be deployed with customers including the NRO Office of Space Launch, Asgardia, Spire, Tyvak, NASA/JPL, and NRL (Naval Research Laboratory) in Washington, D.C. Included in this External Cygnus manifest are also The Aerospace Corporation's AeroCube B/C satellites, water-based propulsion CubeSats.
The External Cygnus Deployment Program was developed with the customer in mind. The lifespan of CubeSat deployed from the Cygnus vehicle at 500 km adds approximately two-years additional lifetime compared to our ISS NRCSD deployment program.
Cygnus Carried the Following CubeSats
• ISARA, a NASA/JPL 3U CubeSat demonstration mission of a Ka-band reflect array antenna.
• EcAMSat (E. coli AntiMicrobial Satellite), a 6U CubeSat of NASA/ARC to investigate space microgravity effects on the antibiotic resistance of E. coli.
• Lemur-2 x 8, 3U CubeSats of Spire Global (commercial global ship tracking). Each of these 3U CubeSats carries two instruments: SENSE, which relays Automatic Identification System (AIS) signals from ships, and STRATOS, which monitors the occultation of GPS satellites as they pass through the atmosphere.
• CHEFsat (Cost-effective High E-Frequency Satellite), a 3U CubeSat of NRL (Naval Research Laboratory) to test COTS technologies.
• Asgardia-1, a 2U CubeSat of Asgardia Space to demonstrate long-term data storage in orbit (500 GB). After leaving the ISS, the robotic Cygnus will boost itself into a 500 km orbit and deploy Asgardia-1.
• OSCD x 2 (Optical Communication and Sensor Demonstration), 1.5U CubeSats of The Aerospace Corporation.
• PropCube-2 (Fauna), a 1U CubeSat of NPS (Naval Postgraduate School) for ionospheric calibration measurements.
• TechEdSat-6, a 3U CubeSat, developed by students of SJSU (San Jose State University), the University of Idaho, and NASA/ARC (Ames Research Center). TechEdSat 6 is the latest in a series of CubeSats testing an "exo-brake" deorbit system, a drag device that uses aerodynamic forces — and not propulsion — to re-enter the atmosphere. The technology could eventually allow some space station research samples to return to Earth sooner, and at less expense.
Cygnus arrived at the station on November 14. Expedition 53 Flight Engineers Paolo Nespoli of ESA and Randy Bresnik of NASA used the space station's robotic arm, SSRMS (Space Station Remote Manipulator System), to capture Cygnus. The crew then handed robotics off to controllers on the ground for the berthing operation. Eventually, Cygnus OA-8 was berthed to Node-1 (Unity, Figure 5). 9) 10)
Two newly arrived CubeSats will be transferred inside the space station to join three others for release into orbit through the Japanese lab module's airlock. These are: EcAMSat and TechEdSat-6.
Cygnus will remain at the space station until December 4, when the spacecraft will depart the station and deploy a total of 14 CubeSats from the NanoRacks CubeSat deployer, mounted on the outside of the spacecraft. Among them are: ISARA, Lemur (8), CHEFSat, Asgardia-1, OCSD (2) and ProCube-2 into a higher orbit of ~500 km. — After these deployments, the Cygnus spacecraft will reenter into Earth's atmosphere and disintegrate over the South Pacific Ocean, as it disposes of several tons of ISS trash.
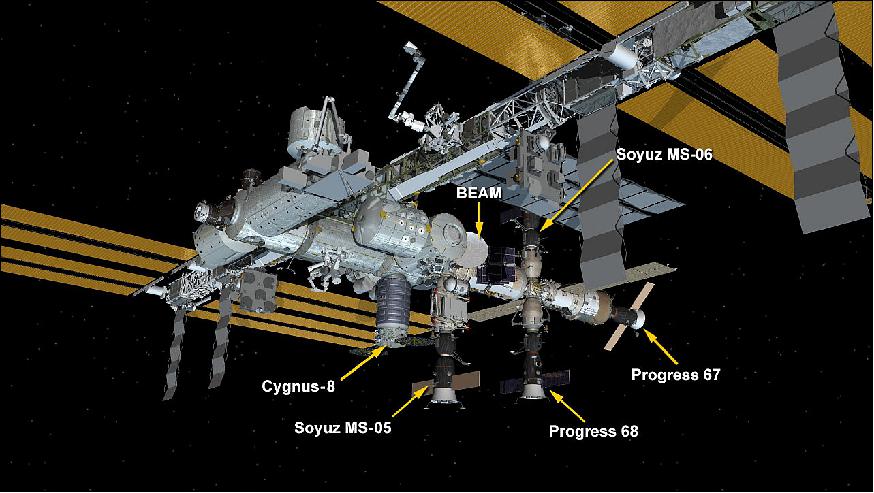
Mission Status
• August 2019: CUMULOS is a three-camera system flying as a secondary payload on the ISARA mission with the goals of researching the use of uncooled commercial infrared cameras for Earth remote sensing and demonstrating unique nighttime remote sensing capabilities. - The CUMULOS payload was activated and achieved first light on 11 June, 2018. Since that time over 120 collection experiments have been executed, over 2000 images have been captured and over a GByte of data downloaded. Much of the mission is automated, with operators involved in target selection, imaging plan generation and anomaly resolution. A target list was compiled which included celestial and terrestrial calibration targets, nighttime lights targets such as large urban areas, maritime areas with high ship traffic or concentrations of fishing vessels, active fires, oil industry gas flares, land cover targets including urban heat islands, snow and ice areas, irrigated croplands, coastal littoral regions, and weather phenomena of interest. Figure 6 illustrates the CUMULOS mission cycle. 11)
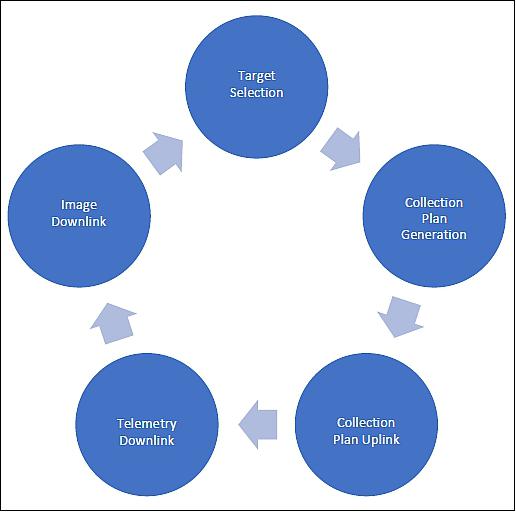
- An Earth-pointed collection experiment begins with operators selecting a target based on location, weather and lighting conditions. Table 1 shows the timeline of an on-orbit image collection experiment. Capturing cloud free imagery of cities was enabled through the use of a weather API and crosschecking with other web-based forecasts prior to tasking. The sequence begins with the satellite nominally tumbling. The attitude determination and control system (ADCS) is initialized only for collection activities. The cameras settings are controlled by sequencing camera register commands to be executed on-orbit.
- Typically the LWIR camera would take a high and low-gain image, the VIS camera would take a sequence of several integration times and the SWIR camera would take a sequence of auto-mode and manual mode settings of high gain, low gain and integration times. The satellite collects data from the three cameras for approximately one minute. During the image collection period the satellite slews to maintain the boresight vector and approximately 20 images are collected with all three cameras. The mission cadence is limited by ground contact availability and the payload downlink bandwidth.
- CUMULOS data is transmitted using the 200 kbit/s UHF radio onboard the ISARA spacecraft. An experimental software defined radio offers the potential for increasing the bandwidth by a factor of up to ten times, but has not yet been successfully demonstrated. A five-station RF ground network is currently operated by The Aerospace Corporation with ground terminals in Florida, Texas, Minnesota, California and Hawaii.
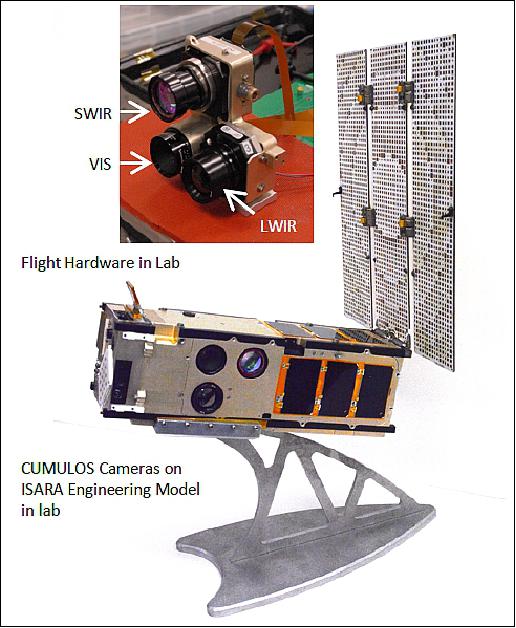
Time | Activity |
t-30 min | System startup |
t-25 min | ADCS initiation |
t-30 sec | Imaging start |
t=0 | Minimum range to target |
t+30 sec | Imaging stop |
t+60 sec | ADCS shutdown |
t+4 hours to 1 day | Ready for next collection activity |
t+ 3days | Image downlink complete |
- Each collection activity requires approximately 2 hours of operator time. Broadly, collection plans fall into three categories - 1) daytime, 2) nighttime, and 3) celestial with camera settings and star sensor collection geometries appropriate for each category. Once the data is downlinked it enters into a data calibration and georegistration pipeline. Work is still in progress to fully automate the data collection and calibration process and the CUMULOS mission is serving as a testbed for this effort with application to current and future prototype remote sensing flight systems. Our collection plan success rate has improved during the mission as our experience with earth sensor, star camera and attitude determination and control system performance improved for different solar illumination scenarios.
CUMULOS Science Targets
- The CUMULOS science target list was divided into a number of different categories: those that enable calibration and sensor studies, and those demonstrate several remote sensing mission areas. These include: stellar, lunar and terrestrial calibration targets, a cities list (for urban geography, growth and change monitoring, light pollution, natural disaster response), severe storms and other weather phenomena, fishing boat areas and other maritime targets, interesting geographical features, and selected satellite conjunction targets (VIIRS -CUMULOS same time collection, often weatherfocused).
- Stellar Calibration Targets: The proper use of stellar calibration sources has been an area of study by Aerospace Corporation astronomers for 20 years or more. We utilize existing stellar models, validated by recent calibrated spectroscopic measurements at astronomical observatories, as our on-orbit calibration standards. The stars chosen initially were αTau (Aldebaran), αLyra (Vega), αBoo (Arcturus), αCMa (Sirius). These stars are bright in both the visible and the short-wavelength infrared and are stable calibration stars, or well-understood and recently measured, as in the case of αTau. Sun-like G-class stars were also considered as calibration targets to reduce the complexity in comparing our results to the solar-diffuser-derived calibration of the VIIRS (Visible Infrared Imaging Radiometer Suite) DNB (Day Night Band). The Sun-like stars that were initially selected proved too dim for us to easily use as calibrators with our small aperture CubeSat cameras. Dark sky targets for dark frame measurements included the north and south galactic poles and the Lockman hole. Star measurements were also used for VIS and SWIR sensor boresight determination, an important step for accurate georegistration.
- The Moon: We take periodic on-orbit observations of the moon at various phases for varying gain and exposure settings using all three CUMULOS cameras. The goal is to gain experience with the use of the empirical USGS Robotic Lunar Observatory (ROLO) model of lunar irradiance. The ROLO model covers 0.35-2.5 µm a range that spans our broadband VIS and SWIR cameras. Comparing results of lunar calibration to stellar calibration is one of our goals, as is the comparison of these results to ground target measurements. We also have used lunar measurements to validate our ground-based flat-field observations. The Moon was also the only celestial target that could be observed by all three sensors in common.
- Terrestrial Calibration Targets: We chose a list of pseudo-invariant ground calibration sites for daytime reflectance calibration, but have not yet taken frequent measurements on these sites, primarily due to our focus on calibrating the nighttime performance of our VIS and SWIR cameras. The sites chosen are from the Landsat test site catalogue. 12) We have started taking measurements of standard thermal radiometric calibration sites used to calibrate the Landsat-8 TIRS and other sensors. Lake Tahoe was the first target selected, and offers a large-area, high altitude, known emissivity, instrumented, uniform LWIR calibration target. Data on this site from multiple lake surface temperature buoys, radiometers and other instruments are published by JPL. 13)
- Urban Targets: Cities chosen for study by the CUMULOS sensors were selected based on size, weather, and coastal proximity. This insured multiple remote sensing phenomenology interest areas could be serviced by one urban imaging experiment. Some of these multi-phenomenology examples will be presented in the thermal sources and weather sections below. All nightlight studies depend on laying down a baseline of data, so our initial cities were chosen to be of general interest to the nighttime lights research community. CUMULOS city data can also be used to better understand the capabilities and limitations of existing VIIRS DNB and DMSP Operational Line Scanner (OLS) data sets and advanced processing techniques. Cities for which imaging experiments have been performed to date include: Abu Dhabi, Baghdad, Hongkong, Istanbul, Jakarta, Jeddah, Kuwait City, Los Angeles, London, Shanghai, Singapore, and Tokyo. Several of these have been imaged multiple times. Weather impacted some collects, making the data more useful for the study of clouds. Many other attempted collections on other cities failed during the time period in which the engineering team was tuning the ADCS pointing, the use of several earth-sensors, and star sensor stray light thresholds.
- Fires, Gas Flares and Industrial Hotspots: The CUMULOS team made an effort to image a number of the severe wildfires that occurred during 2018. The California "Carr", "Camp" and "Woolsey" fires were imaged successfully. Natural gas flares are another subject of interest for remote sensing study for multiple environmental science and economic reasons. Bright flares are also potentially useful as terrestrial point sources for sensor boresight confirmation and georegistration accuracy assessment. Imaging the cities of the Persian Gulf region in Kuwait and the UAE also exhibited a large number of terrestrial and offshore flares, as did Jakarta, Los Angeles and other regions. Other types of flares and industrial hotspots show up in CUMULOS imagery of cities, particularly the SWIR camera.
- Maritime Domain Awareness: Fishing boats are brightly lit at night and are routinely detected by the VIIRS DNB, as are other types of more dimly lit vessels. 14) CUMULOS was tasked to look at areas known to have large numbers of fishing boats, or other concentrations of shipping such as well-traveled shipping lanes. Sites observed with dense ship concentrations included the Java Sea and the Gulf of Oman. Sites were selected in collaboration with Dr. Chris Elvidge's team who create the VIIRS DNB nightly "boat lights" product. The goal is to determine how well a CubeSat can perform this mission and to assess VIIRS performance at detecting densely concentrated ships that can be more easily resolved by our higher resolution CUMULOS VIS camera. Ship lights also appeared in many of our other images of coastal cities.
- Weather Targets: CUMULOS weather targets include: airglow illuminated clouds, severe storms, sea ice, snow-covered terrain and complex cloud scenes in general. A series of on-going conjunction collects between CUMULOS and the VIIRS sensors onboard the Suomi-NPP and JPS-1 satellites, are being performed in collaboration with a team at the Colorado State University Cooperative Institute for Research in the Atmosphere (CIRA) led by Dr. Steven Miller. Goals for this effort include assessing CUMULOS calibration via comparison to the well-calibrated VIIRS sensor, studying the performance of the CUMULOS microbolometer, and comparing the ability of the broadband CUMULOS SWIR camera for airglow imaging of clouds to that of the VIIRS DNB. Demonstrating imaging of clouds at night illuminated by airglow only under zero moonlight conditions was one of the primary remote sensing goal for CUMULOS. We tasked several experiments during new moon with this goal in mind, as well as several conjunction experiments with VIIRS. Additional weather targets are high cold clouds for assessing microbolometer performance. We made an effort to image tropical cyclones during the 2018 hurricane season. Storms on which data were collected included: Lane, Soulik, Rosa, Willa and Yutu.
- Other Terrestrial Targets: Sites with strong thermal contrast signals are of interest, including: land/sea interfaces and littoral regions, urban heat islands and irrigated fields. Some of these overlap with weather imaging interests and include glaciers and snow covered landscapes, sea ice.
- Special Experiments - Laser targeting: The CUMULOS SWIR camera has been used recently as a receiver for laser crosslink demonstrations from space-based CubeSats operated by The Aerospace Corporation. Ground-based lasers are also planning to use the CUMULOS cameras as test receivers for ground to LEO spacecraft pointing experiments. The wide 0.9-1.7 µm band pass make the InGaAs camera a convenient on-orbit laser receiver asset for these tests. The space experiments are described in a paper by Welle. 15)
CUMULOS Celestial Observations
- Observations of stars, the Moon and Mars were taken initially for boresight and focus checks, and then later and more carefully to obtain stellar and lunar calibration data. CUMULOS had a basic boresight check performed in the laboratory on a hot emission point target and laboratory scenes. For initial on-orbit boresight determination, observations were performed on the Moon (visible to all three sensors), and selected bright stars (visible only to the VIS and SWIR cameras). The stars selected were chosen to also be useful for radiometric calibration. We used stellar observations of alpha Tau for accurate VIS and SWIR camera boresight correction. The VIS camera was able to take unsaturated lunar data for some of its settings, as was the LWIR microbolometer. Unsaturated lunar data were used to assess the ground test flat field data, and were also set aside for applying the USGS ROLO model for calibration purposes. The SWIR sensor saturated on the full moon and we were unable to manually program it to take unsaturated data, despite its auto-modes being capable of taking unsaturated Earth scenes. (The auto-mode attempts to boost the integration time and gain for the space scenes since the majority of the frame is dark). Only the register settings needed for nighttime imaging were well-documented enough to be programmed manually by our engineering team. This limitation meant we could only calibrate the SWIR camera for nighttime use. This wasn't a major hindrance since our research goals for this camera mainly involved stellar calibration, nighttime hotspot measurements and nighttime imaging of clouds illuminated by airglow and moonlight.
- Stellar Calibration sources: alpha Tau and alpha Lyra: We chose the two stars, alpha Tau and alpha Lyra, for a series of initial observations to radiometrically calibrate the CUMULOS VIS and SWIR cameras. Stellar spectral flux models were provided by Dr. Richard Rudy of the Aerospace Corporation. Two stellar models are plotted below in Figure 8 along with the solar spectrum for comparison. Alpha Lyra is a hotter star than the sun, and alpha Tau is a cooler star. For both cameras, a sweep of exposure times and gains settings were taken to match and span those used in Earth observation. Data were then dark corrected and flat-fielded using ground test-derived dark correction and flat field files. Example data is shown below from the VIS camera in Figure 9. For the SWIR sensor dithered observations of the stars were performed and the frame pairs were subtracted instead of using ground-based dark measurements. This was necessary to correct for hot pixels which appear in our on-orbit data from the InGaAs focal plane. The stellar radiance signals were integrated within a stellar sampling box, and an outer annulus was integrated and subtracted to remove the residual background. Typical integration box dimensions were 17 x 17 and 31 x 31 pixels for the VIS camera and 9 x 9 and 17 x 17 pixels for the SWIR camera. Results of these flux measurements are plotted in Figures 10 and 11.
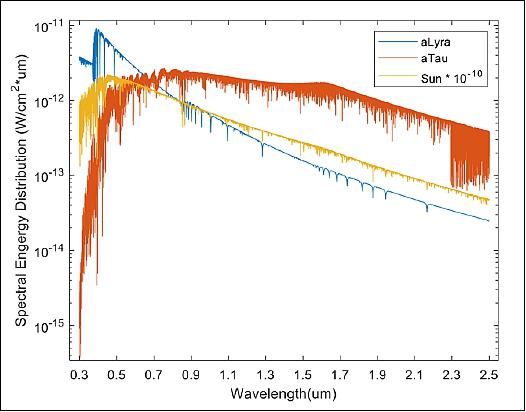
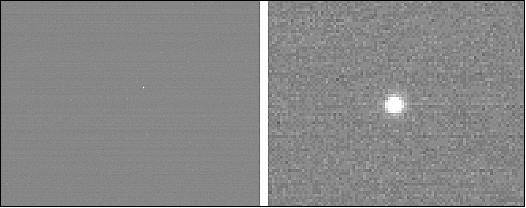
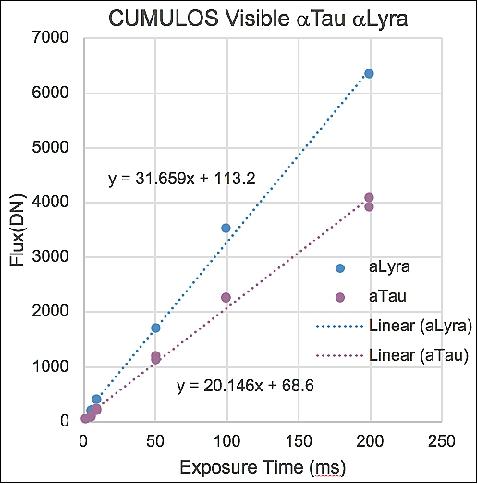
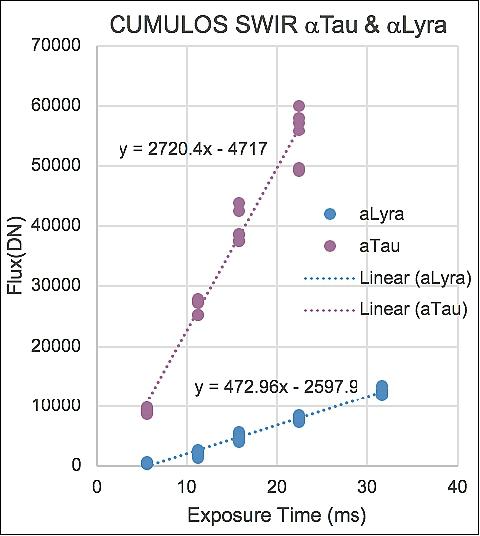
Urban Imaging: Cities at Night
- DMSP OLS and VIIRS DNB imagery are powerful tools for monitoring human development and the human footprint on Earth. These sensors have been used to study human lighting at night for a variety of purposes. These include: mapping light pollution, mapping urban and suburban growth and transportation grids, studying national, regional and municipal energy usage and correlated economic activity, measuring the growth of developing nation's power grids, and mapping post-disaster loss and recovery of power grids. It was the aim of the "nightsat mission concept" to develop a smallsat to address the need to measure nighttime lights at higher resolution, with 20-50 meters as a goal. 16)The CUMULOS VIS camera is a compromise design in a CubeSat form with a GSD of 130 m, and a large enough field of view to study cities and very small countries in a single image. The resolution is a factor of 5.6 improvement over the 740 m resolution of the VIIRS DNB. As a "flying camera" capability in ISS orbital inclination, CUMULOS is similar to ISS nighttime photography. 17) As a free-flying space sensor, CUMULOS is able to image on demand, however, and point freely at stellar and lunar calibration targets for unambiguous on-orbit calibration. The LWIR microbolometer also contributes to the urban imaging mission by providing same-time cloud context imagery for selecting cloud-free data. The SWIR camera adds additional information on industrial hotspots from flares and other hot emission sources. This synergy between cameras also holds for wildfire observations and weather studies presented later.
- Nighttime Imagery of Los Angeles: Los Angeles has been an initial focus of our nighttime remote sensing studies. Our goals are to characterize the regional light emissions and to baseline our CubeSat nighttime lights capabilities for monitoring light pollution at the urban-wildlands-coastal boundaries. The CUMULOS data are currently the highest resolution calibrated nightlights product available for this type of research effort. Understanding these data and using the lessons learned to help build better sensors for future weather or nightlights missions is a goal. Figure 12 shows a 200 ms exposure image of Los Angeles. When imaging cities we take a range of exposures, with 200-500 ms typically providing the best results. A number of features are labeled in Figure 12. The data are logarithmically stretched to highlight both the urban grid and lights from less developed neighborhoods as well as point targets such as ship lights and offshore platforms. Our initial series of images of Los Angeles were taken on the nights of 2018 Oct. 7, 12 and Nov. 13 and allows us to perform some time series comparisons, comparing cloudy vs. cloud-free nights, and also showing the impact on the power grid of the Woolsey fire which impacted the Malibu region. These additional data will be documented in the slide presentation that accompanies this paper. Other targets of interest appear in these related data sets. Our calibrations show we can detect signals as low as 4-8 nW cm-1 sr-1 at typical VIS camera exposure times and noise levels. Empirical comparison to VIIRS DNB suggest we may be able detect signals of 2 nW cm-1 sr-1 as measured by the VIIRS DNB calibration. We will be refining these comparisons as our calibration matures, as conjunction data with VIIRS gets analyzed, and as we reconcile the two sensors different wavebands and calibrations.
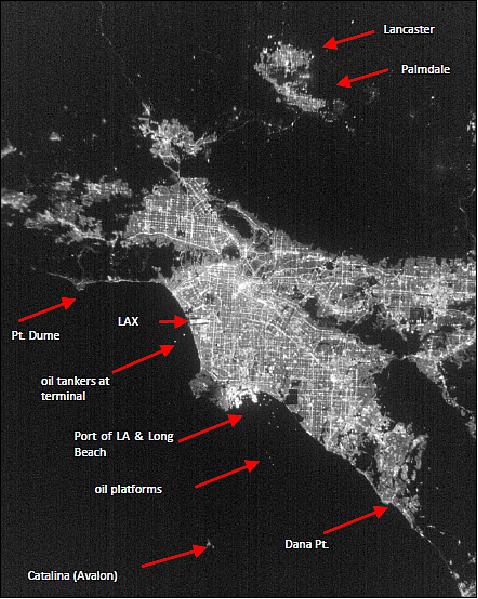
- Figure 13 shows the corresponding SWIR and LWIR camera images. The SWIR image shows extensive urban signals in the 0.9-1.7 µm waveband, but does not map the urban grid as accurately due to its 450 m resolution. Notable SWIR features include hotspots scattered around the region. Some of these are from readily identifiable gas flares, but many others are co-located with landfills and are likely to also be from low-level methane flaring. The LWIR microbolometer image provides cloud context and shows the lack of cloud cover over the Los Angeles metropolitan area. Thermal features from terrain, land-water contrast, including that from a number of warm brightness temperature reservoirs, are evident as are subtle ocean temperature variations. VIIRS DNB data are shown in Figure 14 for comparison. There is no lunar illumination.
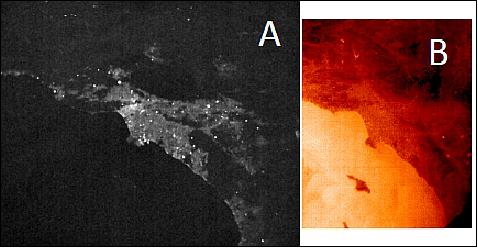
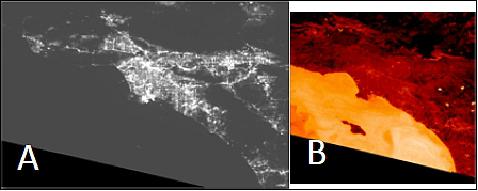
- Two Asian Mega-Cities: Shanghai and Tokyo: Figure 15 shows nighttime data from greater Shanghai and Tokyo side-by-side, major cities with regional populations of 26 million and 38 million people, respectively. A logarithmic stretch is applied to the data to better display the full dynamic range of these nighttime images. Infrastructure such as bridges, roads, airports, rail networks, and refinery flares stand out, as well as subtler lights from ships moored in the Yangtse River and Tokyo Harbor, and dim lights in more remote areas surrounding these two huge cities.
- Shanghai features include: A) Shanghai Hongqiao Railway Station & International Airport, B) Sutong Changjiang Highway Bridge, C) Chongqi Changjiang Highway Bridge, D) Shanghai Changjiang Bridge, E) Shanghai Pudong International Airport, F) Donghai Bridge, G)Yangshan Deep Water Port, H) Hangzhou Bay Bridge. Notable dark areas include the mouth of the Yangtse River, the Huangpu River bisecting Shanghai and Pudong, and Hangzhou Bay.
- Tokyo features include: A) Yokohama Harbor, B) Haneda International Airport, C) Tokyo Harbor, D) JFE Chiba Steel Work, E) Tokyo Bay Aqua-Line bridge/tunnel, F) Chūō Line rapid service rail line showing linear evenly spaced stations, and G) Narita International Airport. Notable dark areas include: Tokyo Bay, though it is full of numerous ship lights and a hint of illuminated haze, Sagami Bay, the Tanzawa Mountain forests west of Tokyo, and the Bōsō Peninsula which forms the eastern edge of Tokyo Bay. The images were both taken at 500 ms exposures.
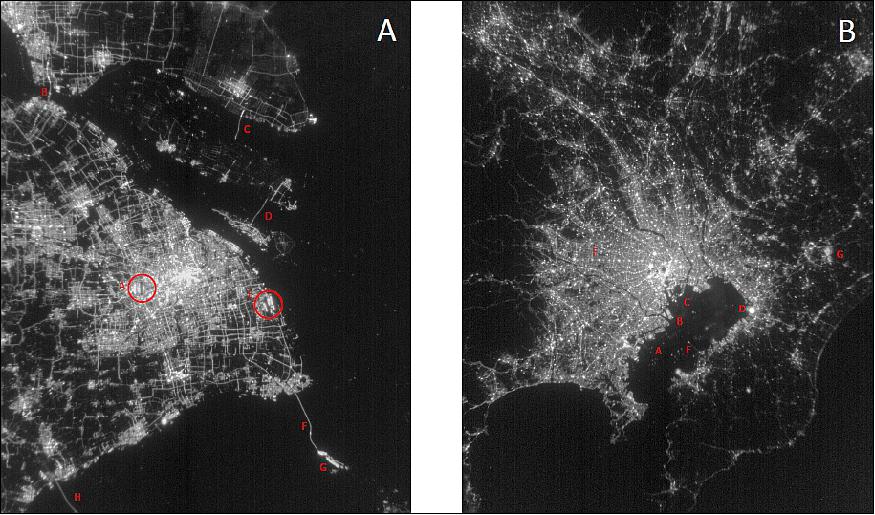
- Interpretation of the VIS camera nightlights data is enhanced by using the thermal and short-wave infrared imagery from the other two camera payloads. Figure 16 shows data from the LWIR microbolometer and SWIR camera for Shanghai. The LWIR camera unambiguously shows the city center is cloud-free with obvious cloud structure far to the north. Other striking thermal features are the Haungpu River bisecting the Shanghai, various lakes, the urban heat island, temperature variations in the river delta zone, and numerous subtle inland canal features. The high gain SWIR image shows waste light in the .9-1.7 µm bandpass from urban lights, bright features from industrial thermal sources such as refinery flares and also images the clouds to the north and east.
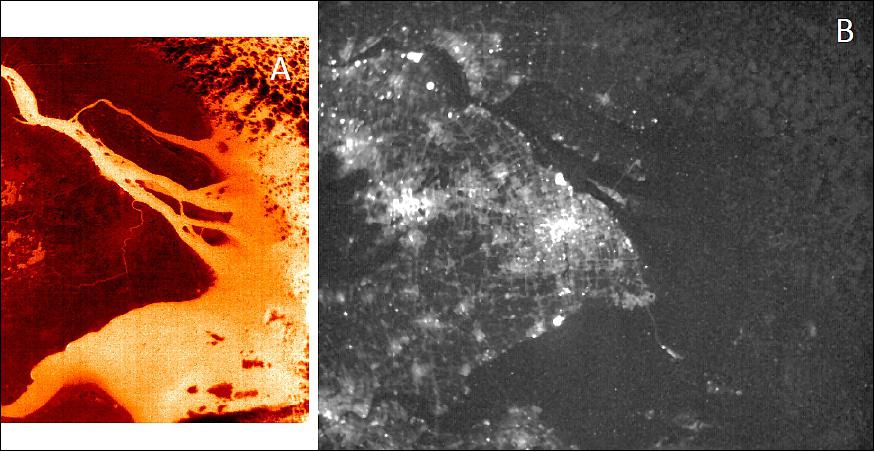
- The complimentary LWIR and SWIR images of Tokyo are depicted in Figure 12. Again, conditions are totally cloud free over the urban area we wish to study. The microbolometer provides excellent thermal context imagery and shows only a small area of scattered clouds out to sea to the southeast of Tokyo and possible haze over Tokyo Harbor. River and lake features appear, as do subtle variations in coastal water temperature. Bright thermal features from a handful of industrial areas are evident in the LWIR only with heavy stretching of the image histogram. The SWIR camera image exhibited saturation in high gain mode on a number of industrial hotspot regions along the edge of Tokyo bay, and on a large refinery complex on the eastern coast of Honshu. The Bōsō peninsula exhibits a notable SWIR hotspot in its otherwise dark center seen in common with the VIS camera. This is possibly from a greenhouse complex. Low warm, clouds are detected in the LWIR over the ocean to the southeast of Honshu, but do not show a strong signal in the SWIR. Other very low signal-to-noise ratio clouds to the east of Honshu barely appear in the SWIR. These example Shanghai and Tokyo imagery show the power of CubeSat-sized sensor systems to provide nightlights, and supporting infrared data over a large urban region. The three sensors work together as envisioned, with the monochromatic VIS camera providing 130 m GSD nightlights, the SWIR data highlighting hot emission sources, and the LWIR data providing thermal cloud cover and terrestrial thermal imagery. When our data pipeline is finalized, these and other examples will be made available with the radiometric calibration applied (for the VIS and SWIR initially), and in georegistered formats. Our goal is not only to provide research quality nightlights data, for the variety of research purposes already discussed, but to develop the process for building, flying and calibrating small spacecraft for follow-on efforts to craft a viable future "NightCube" mission in the spirit of the "Nightsat Mission Concept" paper outlined originally by Elvidge and coworkers (Ref. 16). As we will show in the following section, small staring sensors of the type demonstrated by CUMULOS not only have nightlight mission capabilities, but wildfire monitoring capabilities as well.
Wildfire Observations
The 2018 wildfire season in California had a number of particularly disastrous fires. CUMULOS successfully observed three, the "Carr", "Camp" and "Woolsey Fires". Both the "Carr" and "Camp" fires were observed when they were near their worst conditions. The Woolsey fire was observed after the worst of the conflagration, but showed the impact on the power grid, as well as residual hotspot activity. Figure 17 shows nighttime imagery from the CUMULOS cameras on the "Camp" fire that destroyed the town of Paradise, CA. The SWIR image is a composite of the high- and low-gain images. The three cameras together provide complimentary imagery of the wildfire disaster area with the visible camera detecting the fire emissions and nightlights from nearby communities, the SWIR camera providing the most sensitive measurements of the extent of the fire line, and the LWIR camera detecting the hottest parts of the fire line and providing terrain context imagery. The pattern of the fire line very closely matches that measured by MODIS. 18) CUMULOS results show that building a nighttime-capable fire satellite in CubeSat form is quite possible. To improve overall mission utility and sensitivity, a more capable daytime capability will be desirable to add, either via a MWIR camera, a less solar sensitive SWIR band, or a higher resolution LWIR camera. Explorations of this type are planned for a CUMULOS follow-on study effort, termed AltoCUMULOS, for Advanced CUbesat MULtispectral Observing System.
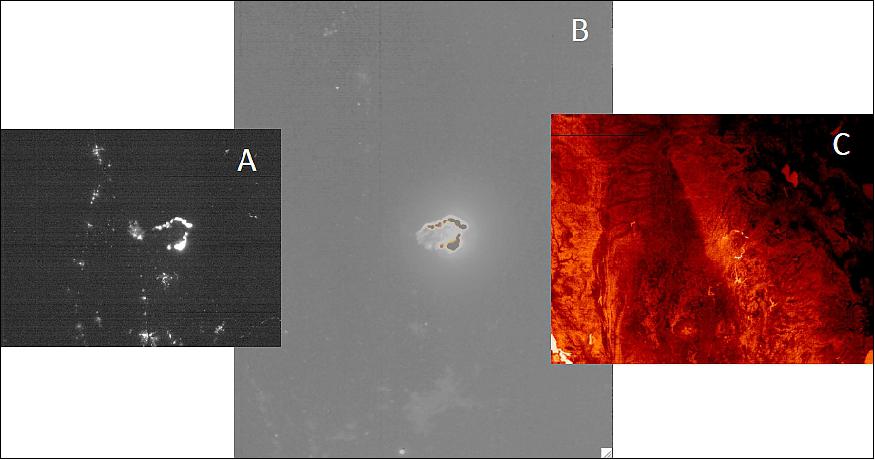
- Georegistration Example - Kuwait City and natural gas flares: Some of the brightest repeatable point source light emitters on Earth are those from large natural gas flares complexes. These make useful fiducial sources for understanding spacecraft sensor boresighting and georegistration accuracy. VIIRS researchers have recently catalogued these emission sources, performed multi-band radiometry on them, and created a useful global map that characterizes global oil industry natural gas flaring activity. We wanted to point CUMULOS at a number of these sites and study sensor performance as well as exploit the measured locations of the flare stacks for georegistration purposes. Figure 18 shows georegistered CUMULOS VIS, SWIR and LWIR data of the Kuwait-Iraq border region which has a number of very bright emissions sources. The Moon was at 12.5° elevation, 236.6° azimuth, and 58% illuminated when these images were taken, and no clouds are evident. The Rumaila gas-oil separation plant flares in southern Iraq appear strikingly in the SWIR imagery. The pushpin and gas pump symbols on the maps mark the VIIRS measured locations of the known frequently observed flare emission sources. Correlating our CUMULOS data with these sites as well as lights from road grids, urban regions for the VIS and SWIR cameras, and land-sea coastal boundaries in the LWIR, allows us to test the accuracy of our autogeoregistration data pipeline. Currently we can georegister data to about 2 pixel accuracy for the VIS and SWIR imagery and are working to improve this and correct the boresight of the LWIR camera to bring its accuracy into line as well.
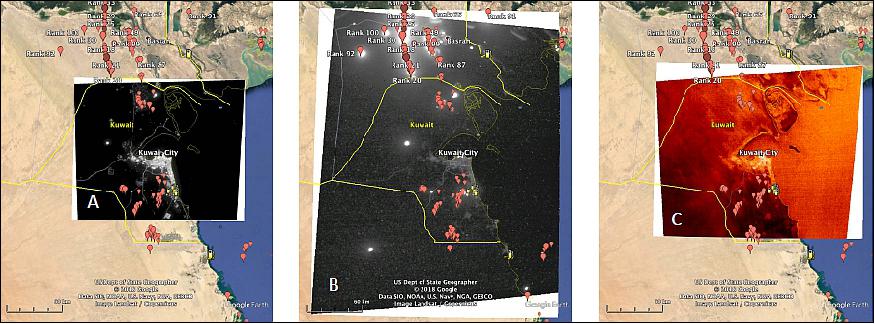
Maritime Domain Awareness
Lights from shipping are routinely observed by DMSP OLS and the VIIRS DNB, particularly the bright lights from fishing vessels which attract fish at night with intense illumination of the ocean surface. VIIRS DNB data are being used by Elvidge and coworkers to generate boat detection products which are being assessed for fisheries management (Ref. 14). 19) Dense concentrations of illuminated vessels can pose a challenge for detection and counting by the VIIRS DNB sensor which has a GSD of 740 m. Figure 19 shows CUMULOS VIS camera data of the coastal border region of the United Arab Emirates and Oman and a map of the region imaged. The data are stretched to enhance the boat light detections. The moon was down at the time of the CUMULOS overpass (moonset was at 19:12 UT). A closely spaced concentration of moored, illuminated vessels, most likely brightly lit oil tankers, are evident off the coast of the port of Fujairah. Another cluster of lights appear to the northeast in the Gulf of Oman just outside the strait of Hormuz as well as other scattered lights from ships at sea. The brightly lit coastal cities appear along with the well-lit highways. A bright signal on the southern coast from an Omani refinery flare appears in all three cameras. Figure 20 shows the corresponding CUMULOS LWIR and SWIR imagery whose larger fields of view touch the Iranian coast. The LWIR shows cloud free conditions on the eastern coast of the UAE and Oman and cooler temperatures at higher elevations inland and possible clouds on the western edge of the image. A thermal source is evident on the coast of Oman co-located with a refinery flare complex. The SWIR camera, tasked in high gain mode, captures the lights from Dubai on the western side of the image, as well as from inland cities on the border of the UAE and Oman, within its larger field of view. It also (barely) detects the ship lights, the coast of Iran and possible low cloud features over the Gulf of Oman (the latter features illuminated by airglow only). There is a stray light artifact in the upper left of the image. We've seen this lens artifact in other images of the Persian Gulf region with very bright emission sources in or near the sensor field of view.
- Other ship light observation experiments have included fishing boat concentrations in the Java Sea, and ships in the Singapore strait, the latter case shown below in the CUMULOS weather observations section.
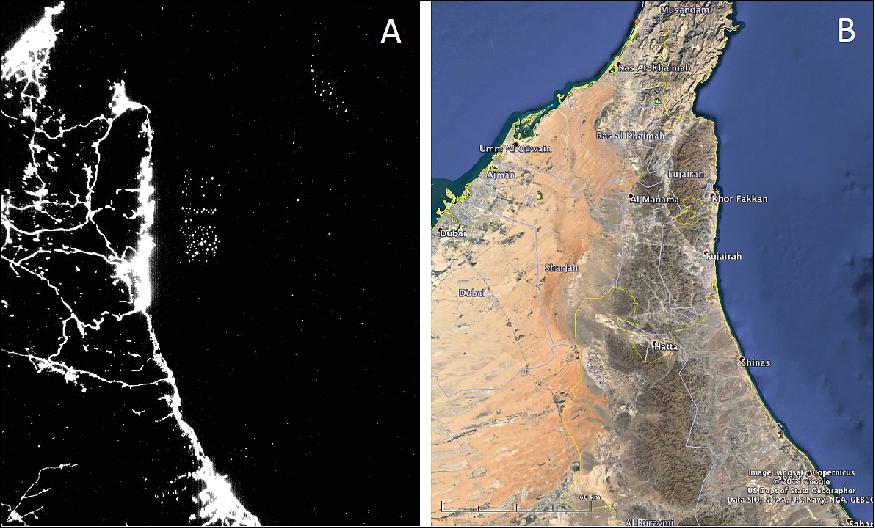
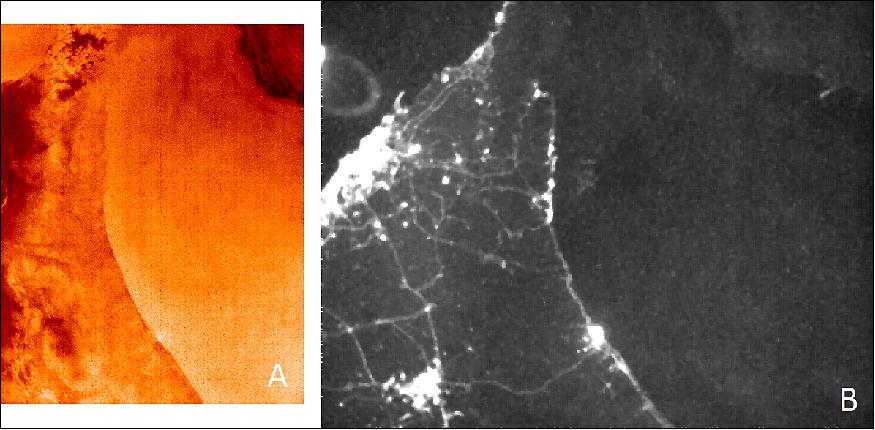
CUMULOS Weather Observations:
We next present a few of our nighttime weather observations, which are also rich in other types of phenomena such as city lights, boats, offshore flares, even an active volcano. We also show new Moon observations of airglow illuminated clouds, including one taken during a VIIRS conjunction. A twilight image of the eye of a tropical cyclone (Hurricane Willa) is also presented.
- Singapore - Moonlit clouds, city lights, ship lights: Singapore exhibits dynamic weather with much thunderstorm activity. Figures 21- 24 show the city state partly enshrouded in clouds, but with some of the city center visible along with moored ships in the Singapore Strait. The necessity of having cloud context imagery when measuring nighttime lights is made plain by images such as these. The moon was at 67.9° elevation, 188.2° azimuth and 74% illuminated. Camera settings were LWIR high gain, VIS 200 ms exposure, SWIR low-gain 32 ms exposure. The nightlights pattern map the growth of the greater Singapore region in Malaysia to the north and in Indonesia's Batam island to the south.
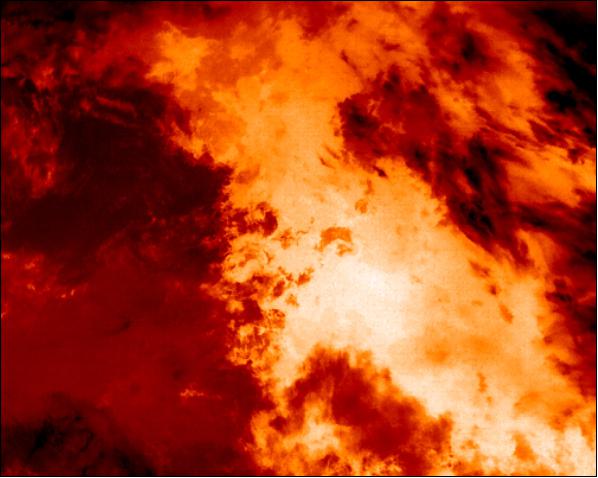
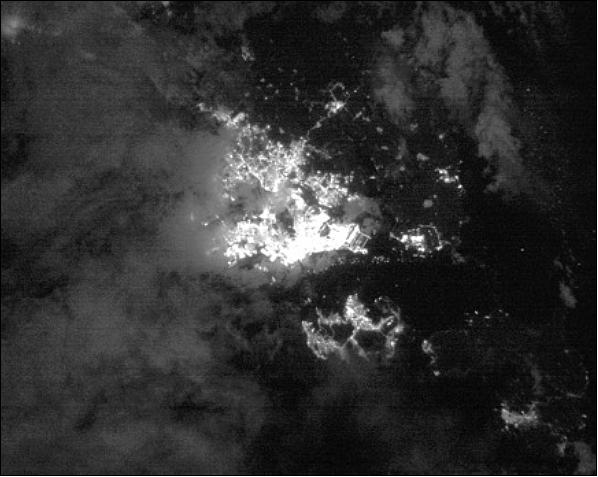
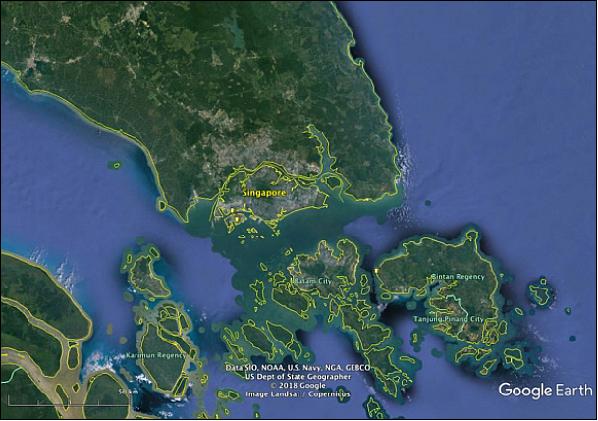
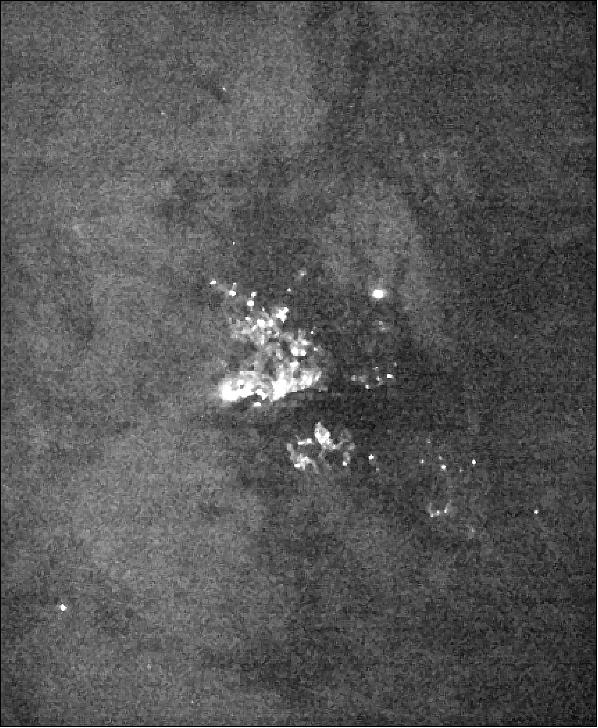
CUMULOS - VIIRS Conjunction, Zero Moonlight
- A series of CUMULOS and VIIRS conjunction collects have been performed. One goal was comparing airglow imaging of clouds from the CUMULOS SWIR sensor to the VIIRS DNB. One such collect was taken over South Australia in the vicinity of Witjira National Park. The scenes are rather featureless except for a bank of clouds that clearly appear in the LWIR data and which are also barely seen VIIRS DNB and the CUMULOS SWIR camera. The CUMULOS VIS camera sees nothing, as the airglow signal is well below its detection threshold. Figures 25 and 26 show the CUMULOS SWIR and LWIR data, and the VIIRS DNB and CUMULOS VIS data, respectively. The median cloud DNB signal is 0.14 nW cm-1 sr-1, right at the edge of detectability for this sensor (non-cloud data values were ~0.1 nW cm-1 sr-1). The CUMULOS SWIR camera data was taken in an autogain mode for which radiometry is not recoverable. Other SWIR frames were taken in programmed modes for which radiometry is possible. These data haven't been successfully reduced yet to extract the calibrated signals which are less distinct than those in the autogain mode image. It is remarkable that the tiny SWIR night vision camera on CUMULOS can detect these weak signals at all with 32 ms exposures. Longer exposures would exhibit higher quality imagery. The airglow signal is the combination of direct to space airglow photons as well as those reflected from the clouds and ground. Taking zero moonlight conjunctions over ocean areas may allow for more straightforward, higher contrast images for comparing VIIRS and CUMULOS results.
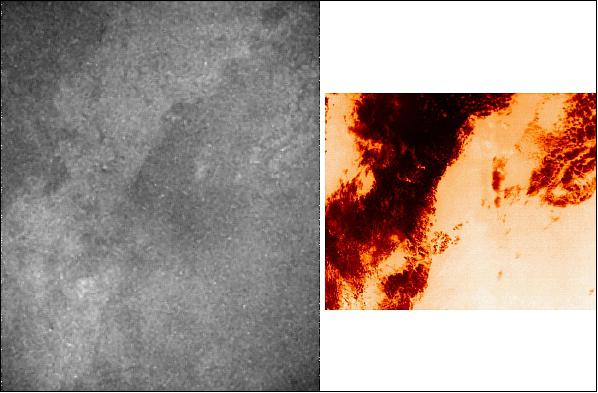
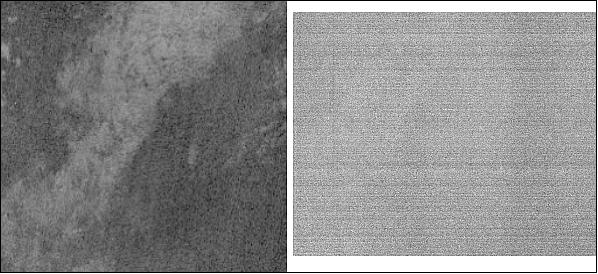
- Hurricane Willa - Twilight Imaging of a Hurricane: CUMULOS was programmed to capture snapshots of the eye of Hurricane Willa off the western coast of Mexico on 23 October, 2018. We wanted to test the microbolometer performance on the highest coldest clouds possible and tropical cyclones are good candidates. The overpass also occurred right after local sunset and over 40 minutes after the last visible geosynchronous image was taken from GOES. Hurricane Willa was reported as a 150 mph, 933 mb category 4 hurricane just before these images were taken. GOES-15 measured brightness temperatures below 60°C in the eye region. Figure 26 shows a LWIR high gain image of the storm center. The sensor is still able to discern cloud structure. This is the coldest Earth-pointed scene the microbolometer has imaged. The 14-bit microbolometer was registering 690 DN on the coldest cloud region. To compare, cold space registers at approximately 620 DN. The microbolometer was losing the ability to subtract its self-emission and detect scene temperature at these levels.
- GOES measured temperatures below 200 K at the same location as depicted in Figure 27, so our microbolometer is running out of signal-to-noise at approximately 200 K in accord with tests in TVAC on the ground. 20) The comparison between the microbolometer data and the twilight VIS camera data shows we're still detecting some valid cloud structure with a microbolometer at these very low cold cloud signal levels. Until issues involving the microbolometer signal offsets are studied further, we're not in a position to perform detailed atmospheric profile modeling and temperature extraction from the CUMULOS microbolometer, but we are gathering data on calibration sites such as Lake Tahoe, as discussed in the following section to further explore its on-orbit capabilities and attempt to better quantify them. Figure 28 shows the CUMULOS VIS camera image. The hurricane eye region cloud structure barely imaged by the microbolometer is clearly seen in this post local sunset image.
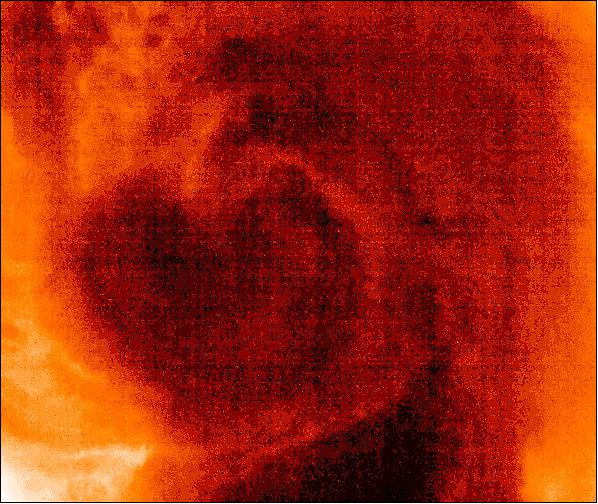
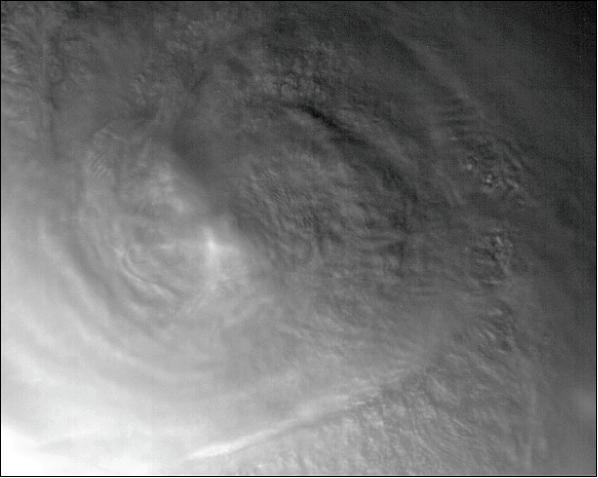
- We used the forecast position of the eye to task the pointing of the CUMULOS cameras to get imagery of the highest, coldest portion of the storm. Figure 29 shows GOES 15 thermal imagery product near the time of the CUMULOS data collection and maps the location of the storm.
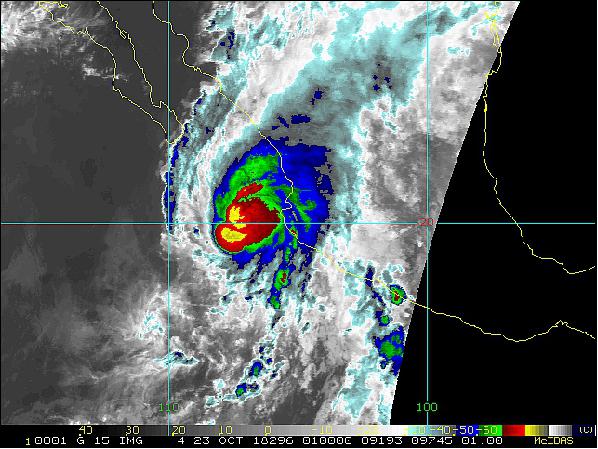
- Daytime Imaging of Thermal Cal Target - Lake Tahoe: Periodically imaging Lake Tahoe under daytime and nighttime conditions for microbolometer calibration studies is an ongoing CUMULOS experiment. Figure 30 shows a largely cloud-free image of the Lake Tahoe region. The CUMULOS VIS and SWIR cameras saturate under many daylight illumination conditions, but can take good early morning and late evening imagery of Earth scenes. The snow covered Sierras appear in this early morning imagery. The SWIR camera shows strong land, water, cloud contrast (with clouds most noticeable in the southwest corner of the image), but no enhanced reflectance from snow. The thermal imagery from the microbolometer highlights temperature differences from the terrain features and highlights Lake Tahoe as a large uniform feature, particularly at the lake's instrumented northern end.
- Other daytime imaging data sets have been taken of snowy terrain showing generally similar results, including Mongolia (in conjunction with VIIRS), far eastern Russia, and the Mt. Everest region in the Himalayas. These data are also being used for testing our automated georegistering data pipeline.
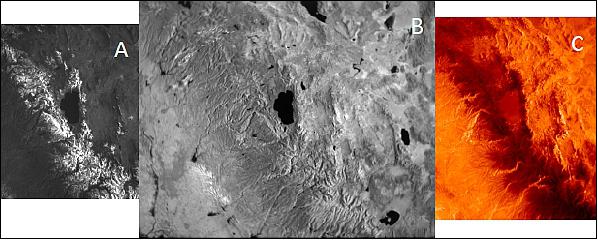
Results (Ref. 11)
CUMULOS was built and tested rapidly to respond to an opportunity to insert a secondary payload on the NASA/JPL ISARA mission. The 3-camera design targeted several "VIIRS-like" missions and has served as a pathfinder for our CubeSat operations and data pipelining efforts. The CUMULOS program has demonstrated several unique contributions during its first year on orbit: stellar calibration techniques, new mission capabilities, and infrared sensor performance in space. These are summarized below.
- Calibration: The CUMULOS project demonstrated the use of bright stars for on-orbit calibration of CubeSat sensors. This technique is suited for radiometrically calibrating the detection of nighttime signals emitted by artificial lighting, fires and gas flares and, if used carefully, can be used to understand the signal levels of moonlit or airglow-illuminated clouds. Other calibration demonstrations are still to be completed, notably implementing the ROLO model using the moon as a VIS and SWIR calibration source, and assessing the performance of the microbolometer through vicarious calibration via VIIRS conjunctions and via modeling and reducing observations of well instrumented thermal calibration sites such as Lake Tahoe.
- High Resolution Calibrated Nighttime Lights: The CUMULOS VIS camera has produced a number of cloud-free observations of major metropolitan areas at 5.6 times higher resolution than the VIIRS DNB. These data can be used to better understand the urban geography, light pollution, energy usage, infrastructure and transportation grids of the studied regions. It is our goal to release these data for general use. In addition to urban areas, experimental observations have been made of gas flare regions, boat concentrations and other phenomena of general interest to nightlights researchers. The SWIR and LWIR data add to the usefulness of these data sets.
- SWIR Airglow-Illuminated Cloud Imagery: The CUMULOS SWIR camera successfully demonstrated imaging of clouds at night in the absence of moonlight, a new approach to the original DMSP OLS and VIIRS DNB mission. By operating in the 0.9-1.7 µm band where the OH airglow Meinel bands peak, we demonstrated that a tiny camera can detect airglow illuminated cloud and land surfaces. These data will be assessed for weather imaging utility, and will be used jointly with the newly operational ISS-based NIRAC (Near InfraRed Airglow Camera) in an effort to better understand the meteorological applications of airglow phenomenology.
- LWIR Microbolometer Utility and Weather Imaging: The CUMULOS program launched the first microbolometer successfully operated in space on a CubeSat. Despite the challenges presented by the orbital thermal environment, the CUMULOS microbolometer has reliably produced clean interpretable thermal cloud context, terrain imagery and hotspot data. Additional work is in progress to fully assess the performance of this FLIR Sys. wide-bandwidth LWIR camera. Performance for cloud imaging degraded at about 200 K, in accord with our ground test data. This doesn't impact the utility of the camera for qualitative cloud cover imaging.
- CubeSat Fire Mission Capability: The CUMULOS program demonstrated nighttime capability for mapping fire perimeters and hotspots via a low-cost three camera VIS, SWIR, LWIR remote sensing system. Performance of the VIS and SWIR sensors was excellent, and produced results comparable to large satellites such as VIIRS and MODIS over the modest field of view of the CUMULOS cameras(Ref. 18). The microbolometer also performed quite well. An operational CubeSat fire mission should have a somewhat higher resolutions if the LWIR is to be used as the primary daytime fire detection sensor, rather than as a cloud context and thermal context imager. This is necessary to increase performance to sub-pixel sized fire lines. Exploring compact MWIR sensors is a goal for a follow-on sensor to CUMULOS.
• April 9, 2019: Two NASA CubeSats teamed up on an impromptu optical, or laser, communications pointing experiment. The laser beam is seen as a brief flash of light close to the center of the focal plane, to the left of Earth's horizon. 21)
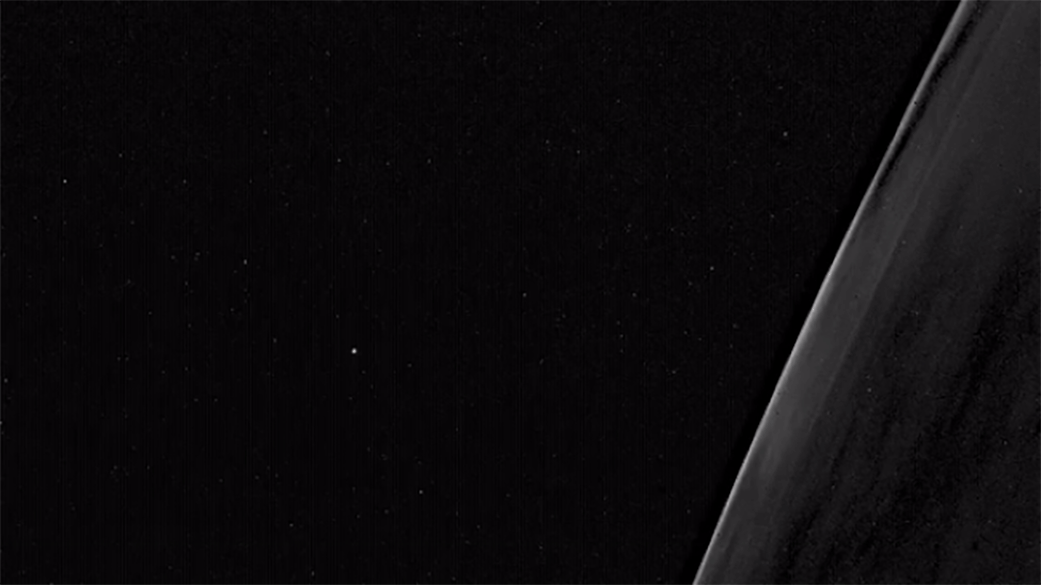
- The optical communications beam was deliberately aimed at and swept across the ISARA camera. This demonstration shows that an optical crosslink between two CubeSats is feasible with proper pointing and alignment of the emitting and receiving spacecraft. Optimizing this capability could enable constellations of small satellites to transfer high volume data between one another in low-Earth orbit or even in orbit around the Moon.
- "This success demonstrates that it is possible to build and operate satellite-to-satellite optical communications links in systems that are substantially smaller and simpler than what's been considered in the past," said Rich Welle, a co-principal investigator for NASA's OCSD mission from Aerospace Corporation. "The future of space communications is optical, and this result can be the first step on a road to making optical communications ubiquitous in Earth orbit, even on the smallest satellites."
- Characteristics built into the design and operation of small spacecraft enable impromptu experiments such as this optical crosslink test. Their flexibility and responsiveness provide mission operators the ability to take advantage of opportunities to perform additional maneuvers and procedures not previously envisioned for a particular mission. Originally designed to be Earth facing, both the ISARA camera and OCSD laser were tipped onto their "sides" to point at one another to accomplish this additional crosslink achievement, an operation much more difficult for larger spacecraft.
- Other features in this image include a star (R Doradus, one of the brightest infrared stars in the sky) that can be seen moving diagonally down toward the right side of the frame as the satellites orbit Earth, and Earth's horizon as it meets space. Other subtle stationary points of white are ‘hot pixels' or digital noise from the camera.
- CUMULOS is an Aerospace Corporation experimental three camera remote sensing payload hosted on NASA's ISARA small spacecraft mission, which was deployed to low-Earth orbit in December 2017. The ISARA mission is managed by NASA's Jet Propulsion Laboratory in Pasadena, California. The OCSD spacecraft were developed and are operated by The Aerospace Corporation. The OCSD and ISARA missions are funded by NASA's Small Spacecraft Technology (SST) program within the agency's Space Technology Mission Directorate.
• August 2018: The ISARA mission has successfully demonstrated a high bandwidth Ka-band CubeSat communications capability that is applicable to commercial, government and military systems. For a modest increase in mass, volume and cost, this technology increases downlink data rates from a baseline of 9.6 kbit/s for existing UHF systems to over 100 Mbit/s – a 105 fold increase in data capacity. The key to this technical advance is a HGA (High Gain Antenna) that is integrated into a commercially available 3U CubeSat solar array with minimal modification of the existing solar panel design. To validate this approach, ISARA has flown a 5 month technology demonstration mission that elevates the antenna technology from TRL 5 to TRL 7 and demonstrates the capability for 100 Mbit/s data rate. 22)
- This system demonstrated 100 Mbit/s downlink data rate capability using a relatively simple ground station with a 70 cm parabolic reflector antenna. The spacecraft also verified ISARA solar panel output power and demonstrated the operational capability to accurately point the antenna to a Ka-band ground station, a key requirement for a telecom system.
- The spacecraft also verified ISARA solar panel output power and demonstrated the operational capability to accurately point the antenna to a Ka-band ground station, a key requirement for a telecom system.
• July 3, 2018: The two images of Figure 32 were acquired with the CUMULOS cameras of the ISARA 3U CubeSat mission. The image on the left, taken by the SWIR (Short-Wavelength Infrared) camera, captures a larger area of the lake and shows strong contrast between land and water features. The narrower field of view image on the right taken by the payload's LWIR (Long-Wavelength Infrared) camera indicates a difference in water temperature between the lake's center and the water in the bays and inlets. 23)
- CUMULOS is testing the performance of commercial sensors for weather and environmental monitoring missions. The cameras are designed for point-and-stare imaging and allow nearly simultaneous coverage of Earth regions from an orbital altitude of 452 km.
- CUMULOS is hosted as a demonstration of an experimental payload on NASA's ISARA (Integrated Solar Array and Reflectarray Antenna), which is managed by NASA's Jet Propulsion Laboratory in Pasadena, California, and operated by The Aerospace Corporation.
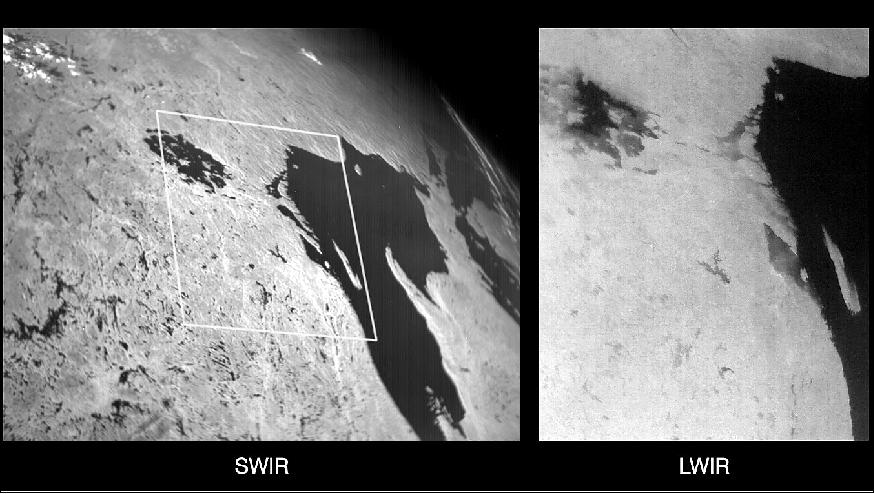
• March 29, 2018: NASA's ISARA and OCSD (Optical Communications and Sensor Demonstration) nanosatellite recently completed systems checkout and have moved into the operational phase to demonstrate a number of technology firsts. 24)
- The ISARA mission is the first in-space demonstration of a reflectarray antenna, as well as that of an integrated antenna and solar array. ISARA is also the first demonstration of the radio frequency Ka-band from a reflectarray antenna. A relatively new type of antenna, the reflectarray consists of flat panels with an array of printed circuit board patches arranged to focus the radio signal in a similar manner as a parabolic dish.
- ISARA initiated demonstration of its radio frequency communications technology by successfully generating a signal tone through its reflectarray antenna to the ground station at NASA's Jet Propulsion Laboratory in Pasadena, California. This demonstration enables high-bandwidth radio downlink of data from a CubeSat-scale spacecraft. The ISARA team will continue to characterize the reflectarray antenna to include measurements related to signal strength and solar array power attainment.
- OCSD consists of a pair of spacecraft each equipped with lower power laser communication systems. Each spacecraft also has a limited water-based propulsion system. OCSD will demonstrate the first-ever high-speed laser communication from a CubeSat to a ground station. OCSD will also demonstrate an optical communications uplink to a CubeSat for the first time.
- Demonstration of OCSD's optical communications payload requires nighttime operations and clear weather, due to the limited power of the laser. During the initial part of this technology demonstration phase, the mission team is working to align each spacecraft's laser with a ground station in preparation for the final demonstration of high-speed downlink optical communications. An optical telescope on Mount Wilson in Southern California will be used for the final demonstration.
- An additional demonstration will involve proximity operations by maneuvering the pair of OCSD spacecraft to within 650 feet of each other. OCSD's proximity operations demonstration requires that the two spacecraft decrease their distance to three miles to enable the laser rangefinders mounted on each spacecraft to locate each other. Currently 100 miles apart, the OCSD spacecraft have fired their water-based propulsion systems to initiate maneuvers to close their distance. Over the coming days, the two spacecraft will approach to a final distance of 650 feet to begin proximity maneuvers.
- The technology demonstrations for both ISARA and OCSD will continue into the summer until completion.
- Managed by NASA's Ames Research Center in California's Silicon Valley, the ISARA and OCSD missions are funded by the Small Spacecraft Technology Program within the agency's Space Technology Mission Directorate. The ISARA payload is being developed by NASA's Jet Propulsion Laboratory in Pasadena, California, and will be demonstrated on a CubeSat developed by The Aerospace Corporation in El Segundo, California. JPL partnered with Pumpkin Inc. in San Francisco, California, to develop the solar array. The OCSD satellites are developed and operated by The Aerospace Corporation of El Segundo, California.
• December 7, 2017: Last night, NanoRacks successfully completed the Company's 4th External Cygnus Deployment mission after commands were sent to the Cygnus spacecraft from Orbital ATK's Mission Control at their Dulles headquarters. After departing from the Space Station, Cygnus was elevated to an altitude of 450 km before deploying the satellites. Note: The Orbital ATK CRS-8 mission was launched on November 12, 2017. - On this External Cygnus Deployment mission, NanoRacks deployed the following 14 satellites: 25) 26)
- ISARA of NASA/JPL
- PropCube-Fauna of NPS (Naval Postgraduate School)
- Lemur-2 (8 CubeSats) of Spire Global
- AeroCube 7 OCSD-B and -C (2 CubeSats) of The Aerospace Corporation
- Asgardia-1 of Asgardia Space
- CHEFSat of NRL (Naval Research Laboratory).
This historic and innovative satellite deployment service is a part of the first-ever program in which an ISS Commercial Resupply Vehicle is able to deploy satellites at an altitude higher than the ISS after completing its primary cargo delivery mission. Flying at 450-500 km provides an open door for new technology development as well as an extended life for CubeSats deployed in low-Earth orbit.
Sensor Complement
ISARA (Integrated Solar Array and Reflectarray Antenna)
ISARA (the payload name is the same as the mission name) is a new type technology providing a very significant reduction in stowed volume. For modest antenna sizes (~30-35 dB gain), the panels can be stowed entirely within the "dead space" between the bus and the launch canister (e.g. P-POD), so the antenna does not consume any payload volume. These antennas are mechanically simple, depending on a simple spring loaded hinge deployment mechanism, have relatively low mass density, and are expected to have low production cost. Perhaps the biggest limitation of the ISARA is scalability. Current technology limits the size to ~3-6 panels due to two factors: (1) tolerance accumulation of multiple hinged panels limits the size of a practical ISARA, and (2) there is a practical limit to how thin one can make a panel and still meet the flatness requirements, so stacking a large number of panels will consume CubeSat payload volume. Nonetheless, the inherent advantages of the ISARA make this a very useful CubeSat HGA technology for many applications. 27)
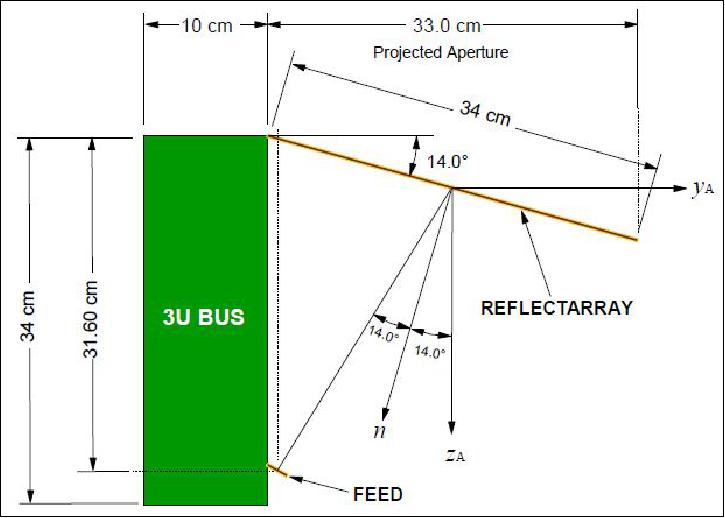
The ISARA design is particularly unique because it incorporates 24 solar cells on the side of the panels opposite the reflectarray, so it can provide both prime spacecraft power and a high speed datalink. The ISARA design is comprised of three 33.9 cm x 8. 26 cm reflectarray panels designed to achieve 33.5 dB of gain at 26 GHz. As illustrated in Figure 33, the feed is mounted on the bus in an offset configuration with a projected aperture of 33 cm x 27 cm. The reflectarray panels are canted 14º relative to the bus so that the specular direction of the main beam is parallel to the bus axis.
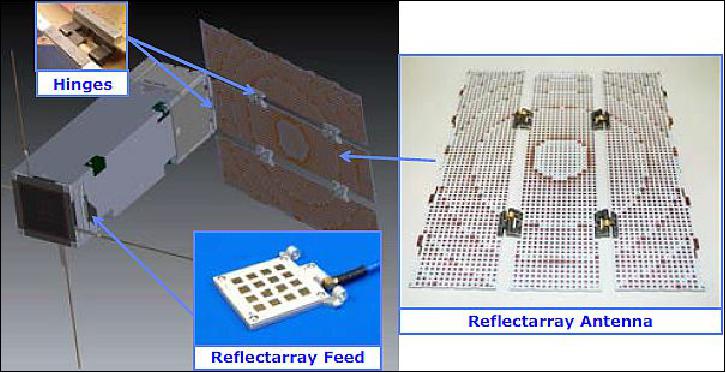
Figure 34 shows a CAD model of the flight configuration along with photographs of the panels, feed and hinges. The deployment mechanism was adapted from the standard Pumpkin Inc. "Turkey Tail" 3U solar panel design. It is planned to make this a commercially available antenna design.
The requirement to maintain panel flatness with expected heating from the solar cells, Earth albedo, etc. drove the design process. Development of a substrate that provides the stiffness to keep the panels flat with the solar cells attached is challenging due the the CTE (Coefficient of Thermal Expansion) mismatch between the solar cells and the panel substrate material. A unique sandwich substrate material was developed using a co-cure process in which 48 mil graphite composite structural core is sandwiched between a pair of 15 mil Teflon based dielectric circuit boards. The outer circuit boards have a dielectric constant of 3.00 and low loss tangent (tan δ ~ 0.001), which is suitable for high efficiency reflectarray panels.
The electrical design of the reflectarray uses square patches arranged on a square grid with an element spacing of 0.46 wavelengths. The feed is a 4 x 4 element microstrip patch array designed to create approximately -10 dB edge taper in order to minimize spillover loss and minimize power incident on the bus. Circular polarization is formed by the feed patch design, whereas the reflectarray is a dual linearly polarized design.
Figure 35 shows the measured principal plane radiation patterns along with the calculated patterns obtained for the EM design. The -13 dB azimuth pattern sidelobes are caused by the large 1.1 cm gaps between the center panel and the two winglet panels, a result of the hinge design used to accommodate the 3U CubeSat bus. Preliminary measurements indicate that the flight antenna will achieve a >33.0 dB gain. The feed design presents one of the greatest challenges for this antenna. The circular polarization requirement imposes practical constraints on the feed array spacing which makes it difficult to achieve an optimal edge taper. In this design, the spillover + taper loss is ~ 2.0 dB. In addition, the requirement to package the feed into a thin, inexpensive printed circuit board package resulted in about 1.4 dB feed loss. Overall antenna bandwidth easily exceeds the required value of 100 MHz.
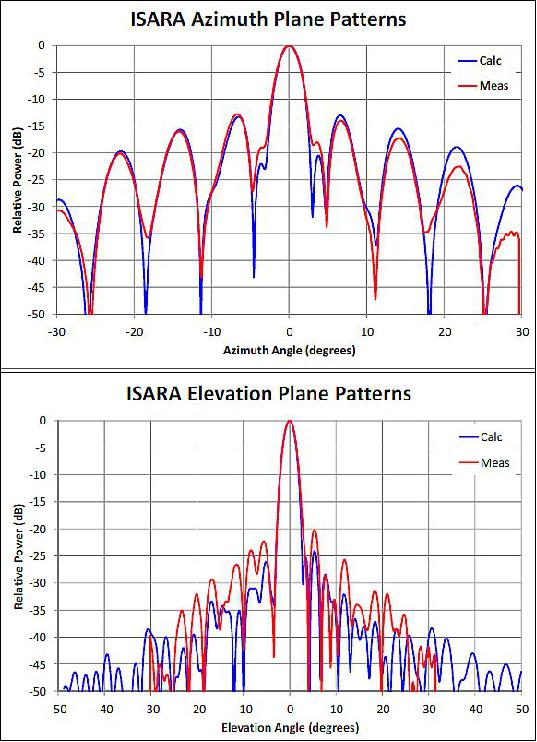
In summary, the ISARA antenna has a great practical value for 3U-6U class CubeSats that need a modest size HGA. The advantages of extremely low stowed volume, low production cost and a low mass perfectly match the CubeSat concept. The ISARA design demonstrates the viability of this concept, although it appears that some improvement in efficiency is possible, particularly in the feed. Nevertheless, the ISARA antenna design scalability is limited by the mechanical restrictions of panel flatness and hinge tolerance accumulation, so the technology is complementary with PRAs (Parabolic Reflector Antennas). Finally, for some missions the option to add solar cells to the reflectarray panel could be very useful.
ISARA Operations
After deployment from the spacecraft, ISARA will deploy its solar array/reflectarray antenna and use the ADCS to de-tumble and stabilize. The UHF system will be used to establish initial communications with the satellite and perform on-orbit checkout procedures. Once the nanosatellite health has been established, on-orbit testing of the Ka-band system can begin.
The Ka-band experiments will include the determination of three elements: data rate capability, antenna gain, and antenna pattern. In order to verify the data rate capability, the received signal will be measured and compared against the estimated receiver noise. The antenna gain will be measured by transmitting a signal and switching between the HGA and the standard gain antenna. Characterizing the antenna pattern involves a multi-pass operations procedure.
CUMULOS (CubeSat Multispectral Observation System)
CUMULOS is a secondary payload on the 3U CubeSat hosting the primary NASA/JPL ISARA mission. The CUMULOS payload was integrated on a noninterference basis and care was taken to ensure low risk to the primary payload mission. CUMULOS will research optimal methods for the operation of passively cooled COTS sensors and cameras and determine their suitability to perform weather and environmental monitoring missions (Ref. 2).
CUMULOS may be thought of as a miniature weather satellite with a special focus on lowlight studies. A primary engineering research goal is to study the use and performance of commercially available uncooled infrared (IR) cameras in space.
Three separate cameras comprise the CUMULOS payload: 1) a visible (VIS) CMOS camera, 2) a SWIR, InGaAs camera, and 3) a longwave infrared (LWIR), vanadium oxide microbolometer. The VIS camera uses an Aptina MT9M001C12STM CMOS chip mated to a ruggedized Schneider Xenoplan lens. The SWIR camera is a FLIR Tau SWIR 25 equipped with a vented lens from Stingray Optics. The LWIR camera is a FLIR Tau 640 using the vendor's lens choice. The visible and LWIR cameras are passively cooled. The SWIR focal plane is temperature stabilized using an internal TEC (Thermo-Electric Cooler).
The cameras underwent TVAC (Thermal Vacuum) and vibration testing independently, and with the integrated ISARA payload. Basic calibration tests such as darks, flat fields, linearity and MTF (Modulation Transfer Function) measurements were performed. 28)
Together, the sensors will be used as a small-aperture, staring payload suitable for testing the performance of passively cooled sensors to perform spaceborne weather and environmental monitoring missions. The sensor specifications are shown in Table 2. The host spacecraft was originally scheduled to be placed in 575 km circular, 97.7° inclination, sun-synchronous orbit with a 10:30 am equatorial crossing time by a Falcon 9 launch vehicle. - Late breaking decisions may enable an earlier launch on the OA-8 ISS resupply flight during Fall, 2017. In this option, after a 6-week dock, the upper stage would then place the host spacecraft into a 500 km circular orbit with 52° inclination. The orbit-dependent terms in Table 1 and Figure 5 will change a bit as a result.
Sensor parameters | CUMULOS VIS | CUMULOS SWIR | CUMULOS LWIR |
Lens f number | 1.4 | 1.4 | 1.1 |
Lens focal length (mm) | 17.6 | 25 | 25 |
Pixel pitch (µm) | 5.2 | 25 | 17 |
Spectral band (µm) | 0.4-0.8 | 0.9-1.7 | 7.5-13.5 |
Data quantization (bit) | 10 | 14 | 14 |
Integration time (ms) | 0.11-900 | 0.18-32 | not appl. 10 ms time constant, 30 Hz max |
Sensor vendor (Type) | Aptima (Si CMOS) | FLIR (InGaAs) | FLIR VOx (microbolometer) |
Array size | 1280 x 1024 | 640 x 512 | 640 x 512 |
Nominal altitude | 500 km | ||
GSD | ~150 m | ~500 m | ~320 m |
Repeat cycle | 14 | 8 | 11 |
Mission concept: CUMULOS is designed to be used as a staring sensor. The basic collection sequence involves taking an image triplet, one frame per camera, as fast as possible to cover a region of interest. Figure 36 shows the pixel and swath of each camera projected onto the Los Angeles region. CUMULOS will obtain near-simultaneous frames with the three cameras. The individual cameras can frame rapidly, but downlink constraints will direct our focus towards taking small numbers of frames from each camera on selected targets of interest. With our current groundstation capabilities we can downlink one to two raw-file image triplets per ground-station contact. During operations, we will downlink thumbnail images and then select which full-frame JPEGs or raw files to retrieve from the 8 GB on-board storage. More full frames may be downlinked if JPEG compression and/or pixel aggregation are used. Use of the SDR radio, currently being tested on AC-7A, increases data downlink rates by a factor of 4. Note that the ISARA antenna is not connected to a radio, so we can't take advantage of its high gain capabilities with CUMULOS.
CUMULOS will leverage the improved pointing capabilities of the current generation Aerospace Corporation CubeSat bus, and add multispectral capabilities, to advance the point-and-stare nighttime imaging experiments we previously initiated with the visible cameras on AeroCube-4, -5, and -8. The three cameras were chosen for nighttime sensitivity and, in the case of the microbolometer, to test an uncooled microbolometer as a cloud and land surface temperature sensor.
Remote sensing applications to be investigated include: cloud detection and characterization, land and water surface temperature measurement, urban heat island measurement, hotspot detection (fires, gas flares, and volcanic activity), and nightlight detection with both the VIS and SWIR sensors. Attempts will be made to image dynamic weather events. While the nominal repeat cycles for all cameras are listed in Table 2, pointing off-nadir will allow more frequent revisit times. Particular attention will be paid to imaging weather at night to research capabilities similar to the VIIRS DNB (Day/Night Band).
The visible and SWIR cameras will be capable of imaging the Earth's surface and clouds when illuminated by moonlight and the airglow. The SWIR 0.9 to 1.7 µm band spans many excited OH emission lines in the atmospheric airglow (the Meinel bands). Much more illumination falls in the SWIR band than in the weak visible emissions detected by the VIIRS DNB under zero moonlight conditions in prior work. 29)
We want to study the utility of the wide-open SWIR band for weather missions and gain experience that can be applied to future weather sensors. The thermal waveband will be used to track cold clouds in the upper atmosphere as well as forest fires on the Earth's surface. Comparisons between bands will aid in performance assessment. Comparisons of CUMULOS data to VIIRS, MODIS (Moderate-resolution Imaging Spectroradiometer), and DMSP (Defense Meteorological Satellite Program) data, as well as data from the Japanese Space Agency CIRC (Compact Infrared Camera) and related on-orbit microbolometers, will be conducted to validate performance.
Calibration verification and on-orbit performance analysis are key parts of the mission goal of testing performance of commercial sensors. Radiometric calibration will be obtained from observations of stars, the Moon, and the Earth, and will be tied to that of other space sensors. Stellar observations with the VIS and SWIR sensors will be used to measure the operational point spread function.
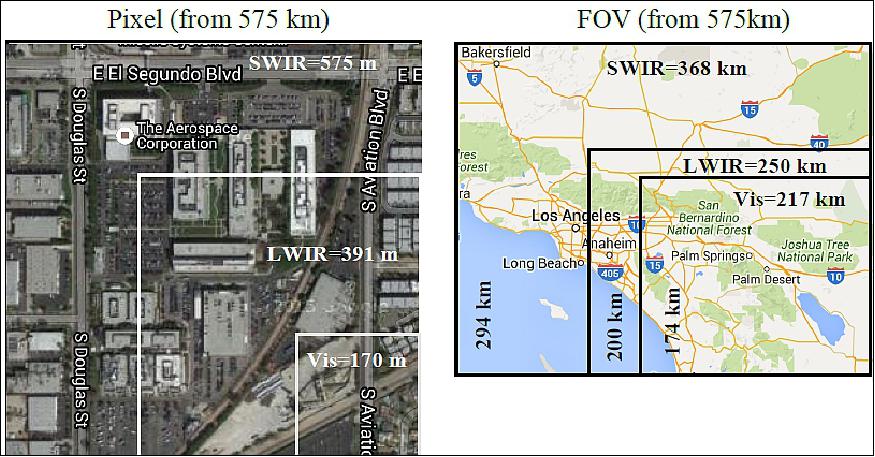
Ground Segment
The ISARA Ka-band ground station, located on top of a nine story building at the Jet Propulsion Laboratory, is the key high data rate experimental link. The station was developed with simple requirements in mind, focused on verifying the Level 1 on-orbit mission requirement following the successful launch. The station utilizes an open loop recording device that allows the team to measure beam peak location, antenna gain and antenna patterns. A simplified block diagram of the station is shown in Figure 37. Since ISARA only transmits a simple carrier wave tone, there is no back-end radio that demodulates "data" (Ref. 22).
To keep costs in line with the project's budget, most of the devices employed in the station electronics are standard off-the-shelf components. The exceptions to this were the positioner, which was a refurbished unit that had recently been declared as excess by the NASA Deep Space Network (DSN), and the 70 cm diameter Ka-band parabolic dish antenna, which was a custom build to accommodate our operating frequency. Additional facilities work was also needed to accommodate the dish in its current location. The resulting station satisfies the requirements of the ISARA mission, but could easily be modified into a more traditional station as needed, leaving JPL with an asset for the future.
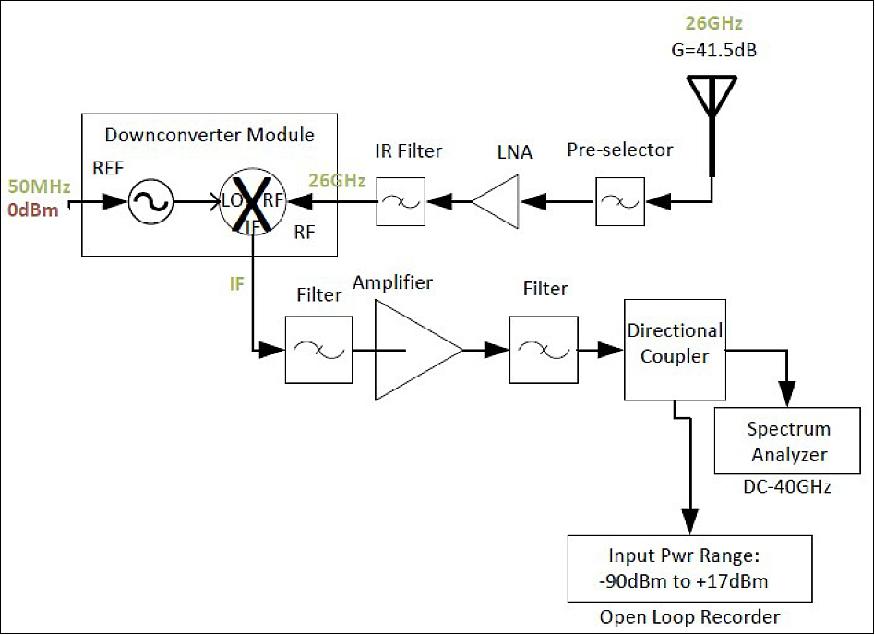
On-Orbit Experiment – Typical Pass: The ISARA CubeSat flies in Low Earth Orbit at 450 km with an approximately 90 minute orbital period and typically a 3-5 minute observation time per pass. In principle, two passes per day are available to measure telecom data rate, gain and antenna patterns. However, in practice it was found that one pass per day was actually usable.
Prior to a pass, the spacecraft and the Ka-band ground station are programmed to execute the required pointing maneuvers and data collection. On the spacecraft side, the ADCS (Attitude Determination and Control System) is programmed to execute the requisite spacecraft pitch, roll and yaw desired for the pass. For example, this could include staring at the ground station, conical beam peak search, pattern cuts to measure sidelobe peaks, etc. The high accuracy ADCS was used to achieve the required 0.2º antenna pointing accuracy. The ground station is programmed to power up and initialize the data acquisition system and point the ground station antenna toward the spacecraft. Experimental passes are operated autonomously and can be run at any time of day or night.
Following a pass, telemetry data is downloaded from the spacecraft through the UHF ground station. This data includes pointing data obtained from the ADCS subsystem, GPS location data and KBE telemetry that includes transmit power level. All of these data are time tagged so that they can be correlated with the ground station recorded data.
Ground station recorded data includes HGA and SGA signal level as well as a record of ground station pointing data. This data is correlated with the spacecraft pointing data in order to verify antenna pointing, plot antenna patterns as a function of angle relative to the spacecraft, etc. Note that during brief periods of a pass the solar array is not expected to generate power. At other times the solar array can be positioned for optimal solar power generation.
Results
The NASA Level 1 requirements for the ISARA mission include both ground measurements and on-orbit results. The purpose of this is to directly verify that the Ka-band antenna on-orbit performance matches what was measured prior to launch. The pre-launch antenna performance will be detailed in a future publication, but is very similar to breadboard data that was reported previously.
The key Level 1 requirement is Ka-band antenna gain. Ground measurements showed the gain is 35.6 dBic, which is in very good agreement with antenna model simulations. The on-orbit passes described above confirmed this antenna gain through a series of conical scans that were used to search for the main beam.
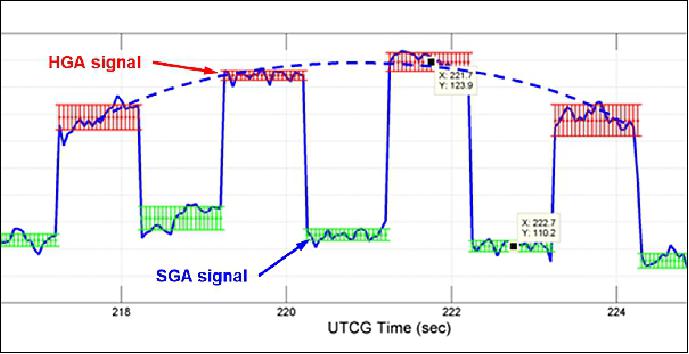
Figure 38 shows signal levels recorded for a typical pass (March 25, 2018). The pulses in the figure correspond to a two second HGA/SGA switching period, so each pulse is 1 second in duration. The HGA signal has uncertainty values highlighted with red shading, while the SGA uncertainty is green. Note the quadratic shape of the high gain antenna main beam is clearly evident (a dashed line has been added to illustrate this). In contrast, the SGA signal level remains relatively constant, which is expected because the SGA beamwidth is much broader.
Averaging several such passes determined a measured on orbit gain of about 32.9 dB with an uncertainty of about 0.8 dB. This value compares favorably to the prelaunch measured gain of 33.6 dB. It should be noted that, due to practical issues that were being solved during the time these data were collected, it is not known exactly how close the measured peak is to the actual beam peak. At least one pass observed a peak gain of 33.4 dB with an uncertainty of 0.6 dB.
These measured gain values have been used in a detailed link budget of the kind JPL routinely uses to predict and assess the performance of spacecraft communications systems. This link budget assumed signal to noise ratio performance values consistent with the ISARA ground station equipment (e.g. a 70 cm ground station antenna). This link budget shows that such a system will achieve a 100 Mbit/s data rate while maintaining an average 5.7 dB link budget margin for a typical LEO mission.
References
1) "Integrated Solar Array and Reflectarray Antenna (ISARA) for High Bandwidth Cubesats," NASA Facts, 2014, URL: https://www.nasa.gov/sites/default
/files/files/ISARA_Fact_Sheet-15Oct14.pdf
2) Dee W. Pack, David R. Ardila, Eric Herman, Darren W. Rowen, Richard P. Welle, Sloane J. Wiktorowicz, Bonnie W. Hattersley, "Two Aerospace Corporation CubeSat Remote Sensing Imagers: CUMULOS and R3," Proceedings of the 31st Annual AIAA/USU Conference on Small Satellites, Logan UT, USA, Aug. 5-10, 2017, paper: SSC17-III-05, URL: https://digitalcommons.usu.edu/cgi/view
content.cgi?article=3613&context=smallsat
3) Dee W. Pack, Brian S. Hardy, "CubeSat Nighttime Lights," Proceedings of the 30th Annual AIAA/USU SmallSat Conference, Logan UT, USA, August 6-11, 2016, paper: SSC16-WK-44, URL: https://digitalcommons.usu.edu/cgi/view
content.cgi?article=3447&context=smallsat
4) "Integrated Solar Array and Reflectarray Antenna for High Bandwidth CubeSats," NASA Facts, FS-2017-03-03-ARC, URL: https://www.nasa.gov/sites/default/files/atoms
/files/isara_fact_sheet-25apr2017-508-cumulos.pdf
5) Courtney B. Duncan, Amy E. Smith, Fernando H. Aguirre, "Iris Transponder – Communications and Navigation for Deep Space," Proceedings of the 28th Annual AIAA/USU Conference on Small Satellites, Logan, Utah, USA, August 2-7, 2014, paper: SSC14.IX-3, URL: http://digitalcommons.usu.edu/cgi/viewcontent.cgi?article=3083&context=smallsat
6) William Graham, "Antares launches with OA-8 Cygnus en route to the ISS," NASA Spaceflight.com, November 12, 2017, URL: https://www.nasaspaceflight.com/2017/11/antares-cygnus-crs-8-iss/
7) "NASA Space Station Cargo Launches Aboard Orbital ATK Mission," NASA, Release 17-087, 12 Nov. 2017, URL: https://www.nasa.gov/press-release/nasa-space
-station-cargo-launches-aboard-orbital-atk-mission
8) "NanoRacks Launches Full External Cygnus Deployer, New Customers, and more to Space Station on OA-8," NanoRacks, 11 Nov. 2017, URL: http://nanoracks.com/launch-of-full-external-cygnus-deployer/
9) Stephen Clark, "Cygnus arrives at space station with food, experiments and cache of CubeSats," Spaceflight Now, 14 Nov. 2017, URL: https://spaceflightnow.com/2017/11/14/cygnus-arrives-at-space
-station-with-food-experiments-and-cache-of-cubesats/
10) Mark Garcia, "Cygnus Installed on Station With New Science Experiments," NASA, 14 Nov. 2017, URL: https://blogs.nasa.gov/spacestation/2017/11/14/cygnus
-installed-on-station-with-new-science-experiments/
11) Dee W. Pack, Christopher M. Coffman, John R. Santiago, "A Year in Space for the CUbesat MULtispectral Observing System: CUMULOS," Proceedings of the 33rd Annual AIAA/USU Conference on Small Satellites, August 3-8, 2019, Logan, UT, USA, paper: SSC19-XI-01, URL: https://digitalcommons.usu.edu/cgi/viewcontent.cgi?article=4447&context=smallsat
12) Landsat calibration activities and research and test sites are catalogued at: https://www.usgs.gov/land-resources/eros/calval, with the test site catalog available at: https://calval.cr.usgs.gov/apps/test_sites_catalog
13) Lake Tahoe test data from a joint JPL/UC Davis project dating to 1999 is catalogued at: https://laketahoe.jpl.nasa.gov/
14) Christopher D. Elvidge, Mikhail Zhizhin, Kimberly Baugh and Feng-Chi Hsu, "Automatic Boat Identification System for VIIRS Low Light Imaging Data," Remote Sensing, Vol. 7, No 3, 2015, pp: 3020.3036, https://doi.org/10.3390/rs70303020, URL: https://res.mdpi.com/remotesensing/remotesensing-07-03020/article
_deploy/remotesensing-07-03020.pdf?filename=&attachment=1
15) Richard P. Welle, Christopher M. Coffman, Dee W. Pack, and John R. Santiago, "CubeSat Laser Communication Crosslink Pointing Demonstration," Proceedings of the 33rd Annual AIAA/USU Conference on Small Satellites, August 3-8, 2019, Logan, UT, USA, paper: SSC19-III-08, URL: https://digitalcommons.usu.edu/cgi
/viewcontent.cgi?article=4402&context=smallsat
16) C. D. Elvidge, P. Cinzano, D. R. Pettit, J. Arvesen, P. Sutton, C. Small, R. Nemani, T. Longcore, C. Rich, J. Safran, J. Weeks & S. Ebener, "The Nightsat Mission Concept", International Journal of Remote Sensing, Vol. 28, No. 12, May 2007, https://doi.org/10.1080/01431160600981525
17) Christopher C. M. Kyba, Stefanie Garz, Helga Kuechly, Alejandro Sánchez De Miguel, Jaime Zamorano, Jürgen Fischer and Franz Hölker, "High-Resolution Imagery of Earth at Night: New Sources, Opportunities and Challenges", Remote Sensing, Vol. 7, 1-23, January 2015, https://doi.org/10.3390/rs70100001, URL: https://res.mdpi.com/remotesensing/remotesensing-07-00001/
article_deploy/remotesensing-07-00001.pdf?filename=&attachment=1
18) D. W. Park, C. M. Coffman, J. R. Santiago, R. W. Russell, "Earth Remote Sensing Results from the CUbeSat MULtispectral Observing System, CUMULOS," American Geophysical Union, Fall Meeting December 2018
19) Christopher D. Elvidge,, Tilottama Ghosh, Kimberly Baugh, Mikhail Zhizhin, Feng-Chi Hsu, Nilo Selim Katada, Wilmon Penalosa and Bui Quang Hung, "Rating the Effectiveness of Fishery Closures With Visible Infrared Imaging Radiometer Suite Boat Detection Data", Frontiers in Marine Science, Vol. 5, 24 April 2018, https://doi.org/10.3389/fmars.2018.00132
20) D. R. Ardila, D. W. Pack, "The Cubesat Multispectral Observing System (CUMULOS)." Conference on Characterization and Radiometric Calibration for Remote Sensing, (2016)
21) "NASA Demos CubeSat Laser Communications Capability," NASA Space Tech, 9 April 2019, URL: https://www.nasa.gov/directorates/spacetech/feature
/cubesat_laser_communications_capability
22) Richard E. Hodges, Dorothy K. Lewis, Matthew J. Radway, Armen S. Toorian, Fernando H. Aguirre, Daniel J. Hoppe, Biren N. Shah, Andrew A. Gray, "The ISARA Mission – Flight Demonstration of a High Gain Ka-Band Antenna for 100Mbps Telecom," Proceedings of the 32nd Annual AIAA/USU Conference on Small Satellites, Logan UT, USA, Aug. 4-9, 2018, paper: SSC18-VI-03, URL: https://digitalcommons.usu.edu/cgi/viewcontent.cgi?article=4104&context=smallsat
23) "Tiny Cameras Snap Pictures of Great Lake," NASA/JPL News, July 3, 2018, URL: https://www.jpl.nasa.gov/news/news.php?feature=7183
24) "Orbital Testing Begins for Advanced Small Spacecraft Communications," NASA, 29 March 2018, URL: https://www.nasa.gov/directorates/spacetech/small_spacecraft/feature/
Orbital_Testing_Begins_for_Advanced_Small_Spacecraft_Communications
25) "NanoRacks Completes 4th External Cargo Ship Satellite Deployment Mission, Largest to Date," NanoRacks, 7 Dec. 2017, URL: http://nanoracks.com/4th-external-cargo-ship-satellite-deployment-mission/
26) "NanoRacks Completes Fourth External Cargo Ship Satellite Deployment Mission, Largest to Date," SatNews Daily, 7 Dec. 2017, URL: http://www.satnews.com/story.php?number=352166275
27) Richard E. Hodges, Daniel J. Hoppe, Matthew J. Radway, Nacer E. Chahat, "Novel Deployable Reflectarray Antennas for CubeSat Communications," 2015 International Microwave Symposium (IMS), IEEE MTT-S, Phoenix, AZ, USA, May 17-22, 2015, DOI: 10.1109/MWSYM.2015.7167153
28) David Ardila, Dee Pack, "The Cubesat Multispectral Observation System (CUMULOS)," Calcon (Conference on Characterization and Radiometric Calibration for Remote Sensing," August 23, 2016, URL: https://digitalcommons.usu.edu/cgi/viewcontent.cgi
?article=1230&context=calcon
29) Steven D. Miller, Stephen P. Mills, Christopher D. Elvidge, Daniel T. Lindsey, Thomas F. Lee, Jeffrey D. Hawkins, "Suomi satellite brings to light a unique frontier of nighttime environmental sensing capabilities," PNAS (Proceedings of the National Academy of Sciences of the United States of America), Vol. 109, No 39, September 12, 2012, pp: 15706–15711, URL: http://www.pnas.org/content/109/39/15706.full.pdf
The information compiled and edited in this article was provided by Herbert J. Kramer from his documentation of: "Observation of the Earth and Its Environment: Survey of Missions and Sensors" (Springer Verlag) as well as many other sources after the publication of the 4th edition in 2002. Comments and corrections to this article are always welcome for further updates (eoportal@symbios.space).
Spacecraft Launch Mission Status Experiment Complement References Back to top