COSMO-SkyMed - Second Generation
EO
Atmosphere
Land
Multi-purpose imagery (land)
The COSMO-SkyMed Second Generation (CSG) is due to be a constellation of 4 satellites, aimed at enhancing the quality and capability of imaging of the original 4-spacecraft COSMO-SkyMed constellation (CSK). The satellites, each with a design life of 7 years, were developed by the Agenzia Spaziale Italiana (ASI) with funding from the Italian Ministry of Defense and the Italian Ministry of Research for both military and civil use. The first two satellites were launched in 2019 and 2022, with the remaining two due to be launched in 2024 and 2025.
Quick facts
Overview
Mission type | EO |
Agency | MoD (Italy), ASI |
Mission status | Operational (nominal) |
Launch date | 18 Dec 2019 |
Measurement domain | Atmosphere, Land, Snow & Ice |
Measurement category | Multi-purpose imagery (land), Vegetation, Landscape topography, Sea ice cover, edge and thickness, Soil moisture, Snow cover, edge and depth, Atmospheric Winds |
Measurement detailed | Land surface imagery, Land cover, Land surface topography, Sea-ice cover, Snow cover, Soil moisture at the surface, Snow water equivalent, Sea-ice type, Glacier motion, Glacier cover, Oil spill cover, Soil type |
Instruments | CSG SAR |
Instrument type | Imaging microwave radars |
CEOS EO Handbook | See COSMO-SkyMed - Second Generation summary |
Related Resources
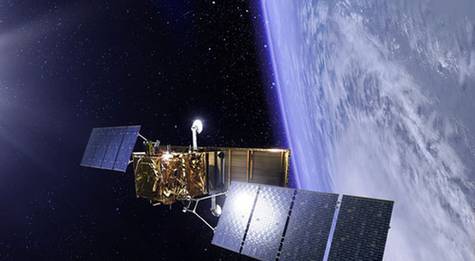
Summary
Mission Capabilities
The CSG system follows the track of the CSK constellation, providing operative continuity to it, but with higher performance goals through advances that enlarge the range of SAR imagery applications. Each CSG spacecraft has a 300% increase in operative bandwidth in comparison to its predecessors. The primary objective is to expand the variety of applications to better cater for forest and environment protection, natural resources exploration, maritime surveillance, cartography and agriculture management.
Each spacecraft of CSG features a COSMO-SkyMed Second Generation Synthetic Aperture Radar (CSG-SAR). The new design has the capability to provide finer resolution than the SAR-2000 instrument onboard CSK, while providing multi-polarization and preserving the observation flexibility. The multi-mode instrument provides a wide range of performance specifications through different implementations of the 3 major SAR acquisition techniques - Stripmap, Spotlight and ScanSAR. In doing so, the instrument fully exploits the range of frequencies allocated by the current ITU (International Telecommunication Union) normative for remote sensing applications in the X-band.
Performance Specifications
The CSG-SAR builds upon its predecessor to provide a broader variety of image properties under the 3 major acquisition techniques. Different management of the polarizations in transmission and receiving of signals give rise to 3 variants of the Stripmap technique: Stripmap, Pingpong and Quadpol, which support a large suite of civilian and defence applications. Stripmap and PingPong are both modes at medium resolution (approximately 3m) which support a swath width of 40 x 2500 km and 30 x 2500 km respectively. Stripmap uses either single or dual polarisation, whereas PingPong alternates different polarizations on a burst basis. Quadpol is an experimental mode using quadruple polarisation with 3m resolution and 15 x 2500 km swath width.
5 implementations of the Spotlight technique have been envisaged in order to achieve various performance figures in terms of resolution, swath width and polarisation. Spotlight-1A and Spotlight-1B have the finest resolution and are devoted to intelligence and defence use. Spotlight-2A and Spotlight-2B have a slightly lower resolution of 0.8m and 1m respectively and a swath width of 10km, in either single or dual polarisation. CSG-SAR is capable of performing the ScanSAR acquisitions in dual polarisation through ScanSAR-1 or ScanSAR-2 modes. ScanSAR-1 mode features a spatial resolution of 4 x 20m and a swath width of 100 x 2500 km, whilst the ScanSAR-2 mode has a spatial resolution of 6 x 20m and a swath width of 200 x 2500 km. All CSG and CSK satellites are positioned in the same orbital plane with a nominal altitude of 620km and complete a circular sun-synchronous orbit with a period of 97 minutes.
Space and Hardware Components
CSG satellites feature a 3-axis stabilisation system with a gyroscope, sun sensors and STT (Star Tracker). It also has a set of 4 reaction wheels, a CMG (Control Moment Gyro) assembly and 3 torque rods as actuators.
The SAR Antenna Subsystem (SAS) compounds the majority of technology and design innovations that characterise the CSG mission. The SAR antenna modular design consists of ten identical “macro tiles”, with a high degree of electronics integration and density. Each macro-tile can manage signals at a very large pulse bandwidth and simultaneously receive radar signals in H and V polarisation. Each macro-tile contains double the number of TRMs (Transmit Receive Modules) with respect to CSK, on a surface of the same dimensions, along with service and control units. Each spacecraft has a mass of 2230 kg and a design life of 7 years, with 24 hours of operative autonomy.
COSMO-SkyMed Second Generation (CSG) Constellation
Space Segment Launch Mission Status Sensor Complement Ground Segment References
Italy's second generation COSMO- SkyMed constellation of two satellites, also referred to as CSG (COSMO-SkyMed Seconda Generazione), aims at improving the quality of the imaging service, providing the end users with enhanced capabilities in terms of higher number of images and image quality (larger swath and finer spatial and radiometric resolution) with respect to the current COSMO-SkyMed constellation, referred to as CSK. - The CSG requirements call for additional capabilities (e.g. full polarimetric SAR acquisition modes) granting a greater operative versatility, both in terms of programming capability and the effective sharing of the system resources among different typologies of users requesting images of different characteristics.
The CSG mission has been conceived, according to the requirements stated by ASI (Agenzia Spaziale Italiana) with funding of the Italian Ministry of Defense (I-MoD), at the twofold need (civil/defense) of ensuring operational continuity to the currently operating “first generation” CSK constellation, while achieving a generational step ahead in terms of functionality and performances. In order to ensure operational continuity, the new CSG satellites will be ready for operations in time to replace the CSK satellites whenever they are being progressively phased out at the end of their lifetime, expected to start from 2015 onwards. 1) 2) 3) 4) 5) 6)
With the aim to guarantee the operational continuity of the current COSMO-SkyMed constellation and to consolidate the excellent scientific and technological know-how up to now reached by Italy in the SAR field, ASI awarded TAS-I (Thales Alenia Space Italia) in 2011 the phase-B contract for the CSG program.
Overall, the CSG system follows the track of the CSK constellation, providing operative continuity to it and maintaining its main characteristic of dual-use, but with higher performance goals through technology advances that enlarge the range of SAR imagery applications.
CSG is based on a top level, state-of-the-art technology with the following guiding principles for the evolution of system implementation:
• A brand-new design of the SAR (Synthetic Aperture Radar) instrument, capable to make the space resolution of the “narrow field images” finer than CSK, while providing multi-polarization. This involves in particular the design of the SAR central electronics and active phased array antenna with respect to CSK 1st generation.
• A new PDHT (Payload Data Handling and Transmission) design that significantly improves the performances of the PDHT currently in use in CSK, in terms of on-board data storage capacity (doubled), space-to-ground data transmission throughput (doubled), data reception rate from SAR. The innovations relate to all elements of the PDHT, such as on-board memory cells, command & control software, data encryption, modulation scheme, communication devices.
• A satellite platform enhanced from CSK, in terms of augmented electrical power (i.e. more than 40%, necessary to sustain the imaging performances), a new state-of-the-art design of AVS (Avionics Subsystem) in support for very effective satellite agility, and an increased propulsion fuel tank capacity for a prolonged operative lifetime.
• The CSG ground segment will be upgraded upgraded from the CSK implementation to complement the CSG space segment innovations necessary to manage, control, and utilize a system with an increased performance. However, the architectural integration between the ‘old’ CSK and the new CSG ground architectures must be ensured. The CSG ground segment will implement increased IEM (Interoperability, Expandability and Multi-mission/Multi-sensor) operations.
• The ILS&OPS (Integrated Logistic Support and Operations) segment. ILS&OPS is a CSK and CSG operative perspective, providing a unified suite of tools and procedures for managing, operating, and maintaining the integrated “COSMO system” that will govern both constellations.
With the deployment of two new satellites and the evolution of the existing ground infrastructure, CSG will provide an improved product quality to satisfy the user requirements: CSG will fulfil new and more demanding requirements in terms of response time, new products, security rules, data confidentiality, quality and number of images per orbit and per day.
The suite of different image topologies and characteristics supported by the COSMO Second Generation, compared with CSK, are shown in Figure 1.
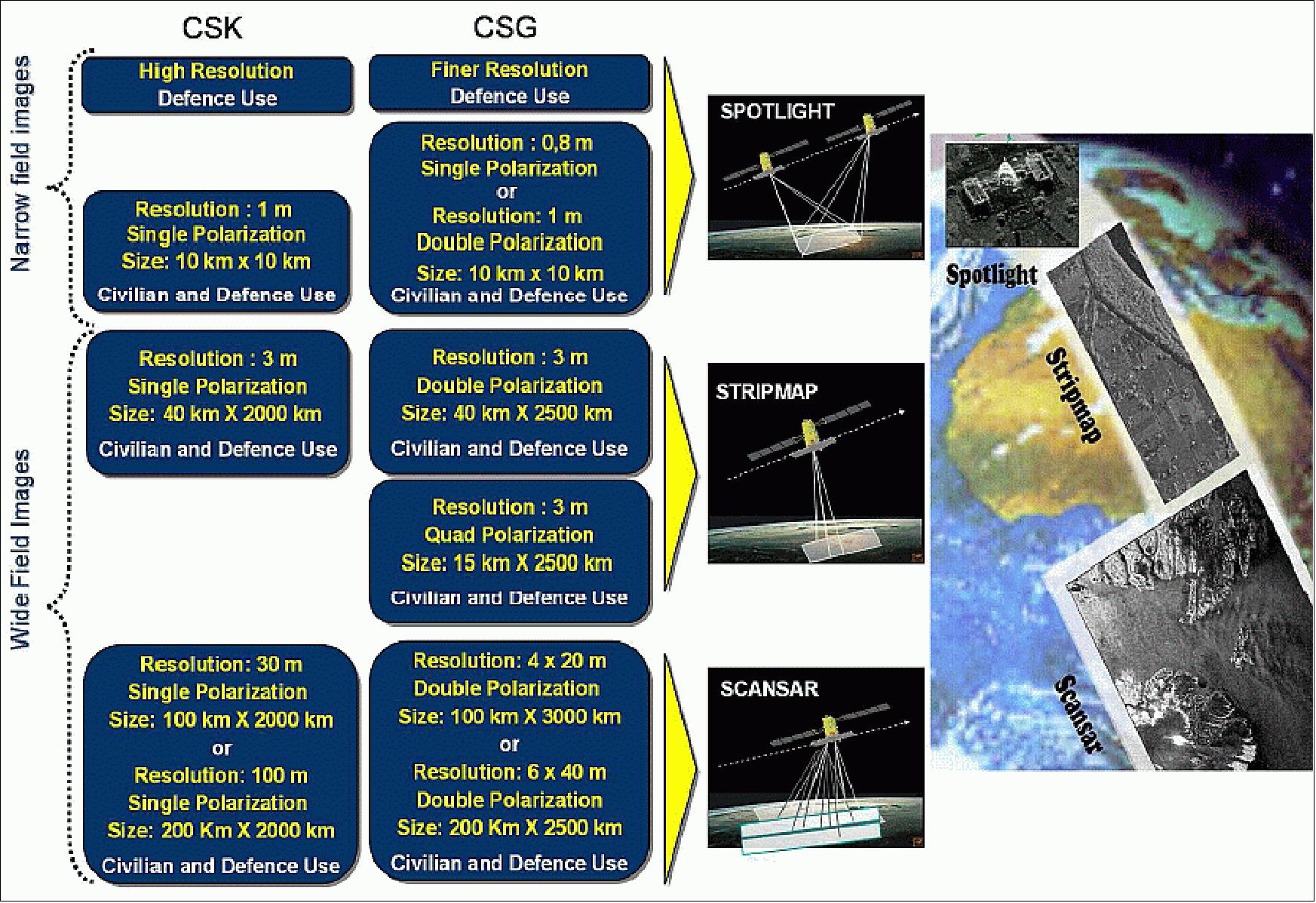
Spacecraft
The CSG program is funded by the Italian Ministry of Research and Italian Ministry of Defence (I-MoD) and managed by the Italian Space Agency (ASI) in conjunction with I-MoD. The satellite represents the state of the art of LEO spacecraft medium size technology and is composed by the following main architectural components: 7) 8)
• PRIMA platform: CSG platform is based on the PRIMA concept which foresees three main modules structurally and functionally decoupled to allow the parallel modules integration and testing activities up to the satellite final integration. The modules are: the service module carrying only bus units apart from the propulsion ones; the propulsion module (enclosed into the service module) carrying all the propulsion items connected by the pipelines and the payload module carrying all the payload equipment including the pertinent appendages.
• EPS (Electrical Power Subsystem): The EPS guarantees to cope with the high peak power required by the payload (18.6 kW) for the whole operational life (7 year). The subsystem provides power through solar arrays in sun-light and through the battery in the eclipse phase. The bus voltage operates at 65 V for the unregulated bus voltage and 28 V for the regulated one. During the SAR payload imaging, the battery provides to the antenna, through a dedicated supply SPDU (Switched Power Distribution Unit), the power in excess wrt the solar arrays output. The electrical power subsystem also foresees opportune protections against short circuits or anomalous power absorption.
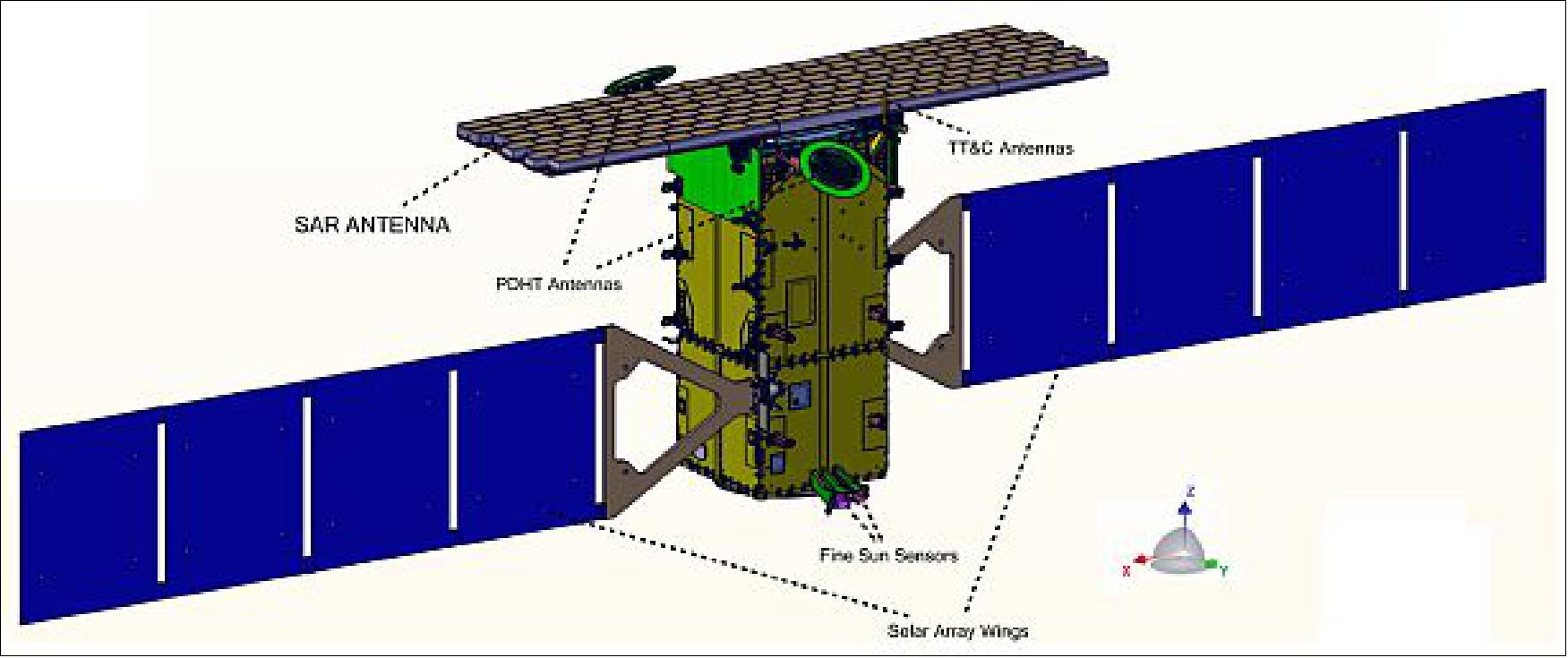
AVS (Avionics Subsystem): The AVS features gimbal-mounted CMGs (Control Moment Gyros) as actuators to provide maximum agility of the spacecraft. The SMU (Spacecraft Management Unit) is the core of the avionics system and controls the satellite operations; it provides attitude and orbit control; collects, stores, manages and downlinks telemetry; receives, stores, manages and executes commands; maintains and distributes S/C On Board Time; provides synchronization services, and is responsible for satellite FDIR (Failure Detection, Isolation and Recovery). The units that constitute the AVS are the SMU, sun sensors, gyro, magnetometer, star tracker, GPS receiver, magnetic torque rods, reaction wheels and CMG assembly. The CMG assembly permits to greatly improve spacecraft agility allowing to operate in spotlight and stripmap modes with a constant pitched attitude both in left- and right-looking and to perform left-right looking maneuvers (and vice versa) in less than 4 minutes (including stabilization).
• The spacecraft features a 3-axis stabilization system with gyro, sun sensors and STT (Star Tracker), a set of 4 reaction wheels, a CMG (Control Moment Gyro) assembly and 3 torque rods as actuators, with steering capabilities on each axis, high pointing accuracy and knowledge and real-time orbit determination.
• A Mil-1553 command bus as computer, avionic and satellite communication backbone and Leon 3 processor architecture. The spacecraft is capable to largely simplify ground operations by providing on board a powerful commanding tool and a large capability for command storing to achieve up to 24 hours of operational autonomy.
• A monopropellant propulsion system with 154 kg of hydrazine and dual branch with 6 thrusters.
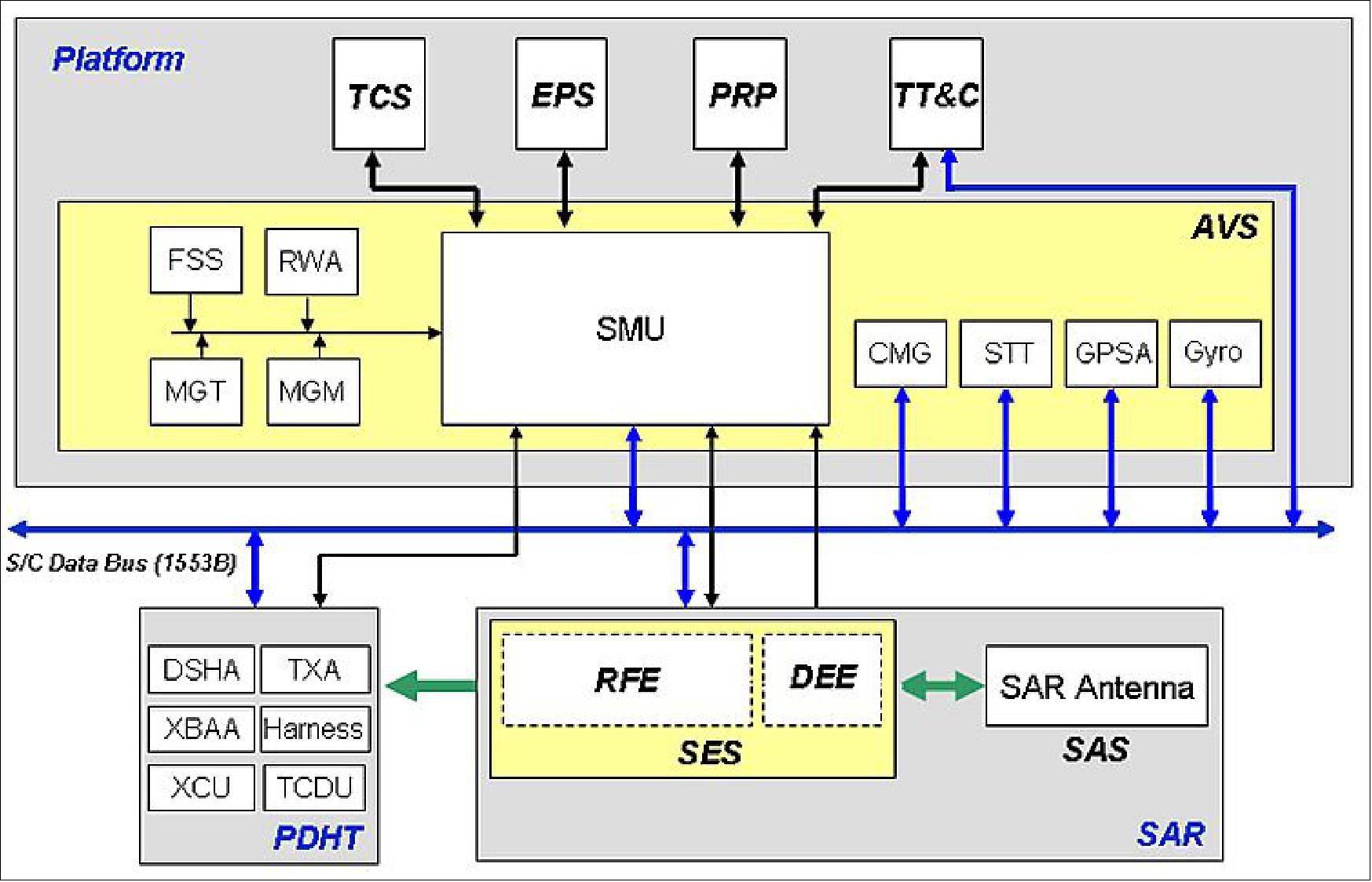
PDHT (Payload Data Handling and Transmission) architecture: The PDHT comprises all functions necessary for the real-time acquisition, storage and handling of SAR data generated by the payload, and for their transmission to the ground station.
The PDHT instrumentation is composed by following main assemblies / units: 9)
• DSHA (Data Storage and Handling Assembly): the objective of DSHA is to provide the functions of acquisition, storage, formatting, encoding and encryption of payload and auxiliary data and to command and control of all PDHT equipments.
• XCU (X-band Crypto Unit): the objective of XCU is to provide the function of ciphering of the acquired data for dual use applications.
• TXA (X-band Transmission Assembly): the objective of TXA is to provide the functions of encoding and modulation of the baseband data stream received from the XCUs, and to power amplify and filter the modulated signals to be transmitted on the X-band link.
• XBAA (X-Band Transmission Assembly): XBAA is required to transmit the X-band signals to the ground, according to the defined performance, supporting the spacecraft right and left looking modes.
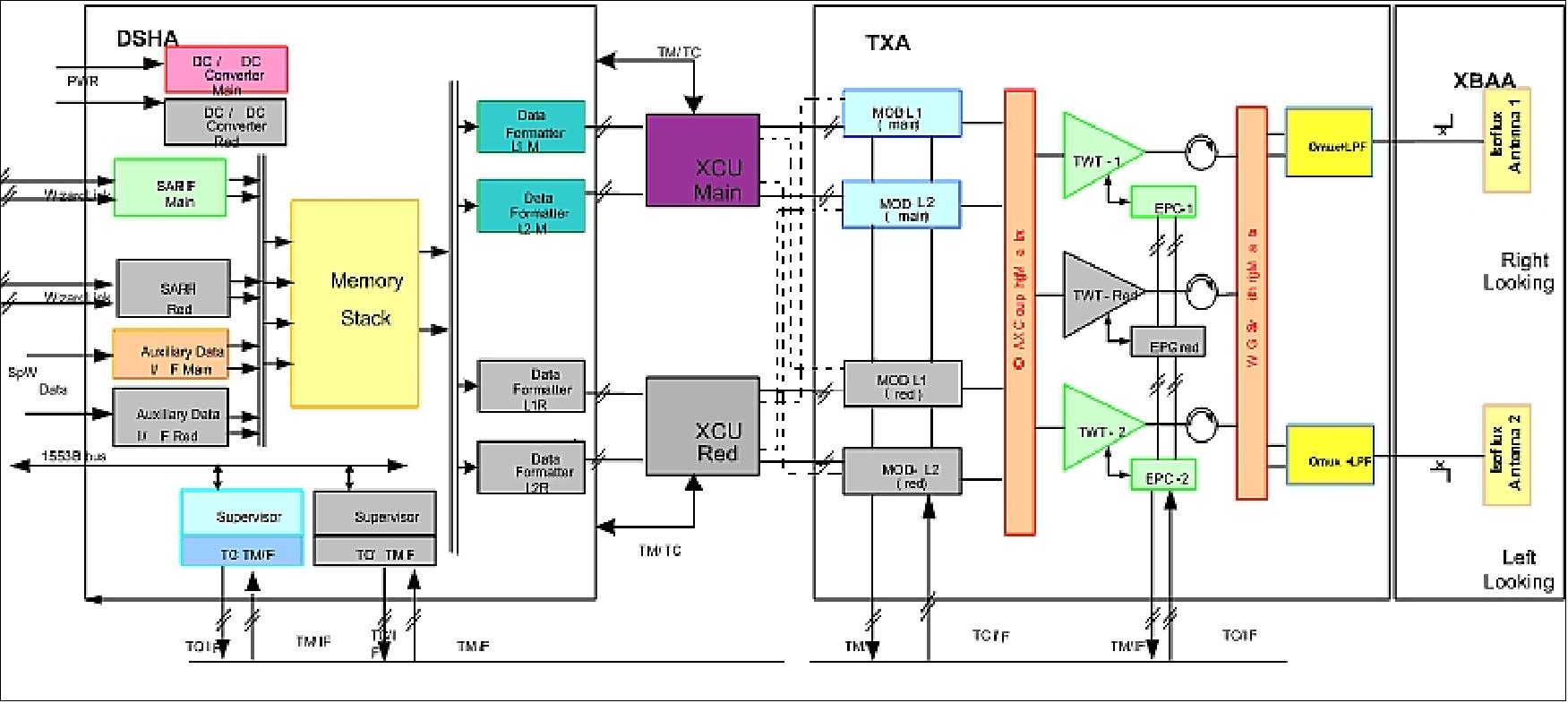
DSHA: The CSG-SAR payload interfaces at the DSHA input are implemented with new physical I/Fs instead of the Hotlink transceivers used on the CSK satellites. SAR acquisition data rates up to 2 x 1.2 Gbit/s, and the need to contain the number of interconnection harness, are the design drivers for the selection of the transceiver to be used: The WizardLink I/F has been selected, considering that this component is already qualified; it sustains very high data rates to comply with the CSG needs.
The DSHA assembly within the frame of the CSG program is required to support continuously very high storage and data handling performances. For the mass memory implementation, the identified solution makes use of higher capacity memory modules. Fortunately, the reduced size of these new high capacity modules allow to accommodate the DSHA into a single box architecture - featuring an implementation with lower power consumption, lower mass, and with a reduction of required harness w.r.t. the dualbox approach in CSK. The DSHA memory architectural configuration will make use of the SDRAM technology.
The on-board memory capacity provided by the single DSHA box is dimensioned to ensure the storing of images very demanding in terms of size, allowing the proper total PDHT storage capacity at End of Life (required by CSG mission: 1530 Gbit, EOL). In terms of input/output performances, the DSHA will be able to handle the concurrent storage of two SAR data files received through two independent Wizard links, supporting in this way an overall input data rate up to 2400 Mbit/s and a gross output data rate up to 560 Mbit/s in downlink using two channels of 280 Mbit/s each.
A civilian encryption functionality it is also provided by DSHA for each of the two output data streams. This will ensure the confidentiality of the observation data for the civilian users.
The auxiliary data (e.g. GPS raw data) will be received through a dedicated interface implemented through a SpaceWire I/F. Telecommands and telemetry will be managed as PUS (Packet Utilization Standard) compliant packet.
TXA: The design improves the downlink performances by introducing an 8PSK modulation scheme with proper channel coding. The most suitable coding scheme is represented by the 4D-TCM combined with a Reed Solomon since its code gain is really helpful in the X-band transmission link establishment and maintenance.
SAR antenna charachterizing parameter | CSG (COSMO Second Gereration) | CSK (COSMO-SkyMed) |
SAR antenna sizes | 5.7 m x 1.4 m | 5.7 m x 1.4 m |
Spacecraft mass | 810 kg | 600 kg |
Absorbed power | 17 kW | 11 kW |
RRF peak power | 9 kW | 5 kW |
Operative bandwidth (% of increase) | 300% CSK reference | CSK reference |
Polarization | Double or quadruple simultaneous polatrization | Single selectable |
SAR antenna electronic structure | 10 macro tiles | 40 tiles |
Number of T/R modules | 2560 | 1280 |
| Chip HPA in pHEMT (pseudo-morphic High | Chip HPA in BJT (Bipolar Junction Transistzor) |
Core chip in Enhanced Depletion Mode | — | |
Number of power suppliers | 40 | 80 |
Number of digital controllers | 10 | 40 |
RF calibration | Active through core chip | Through passive path |
Spatial resolution (NIRSS grade) of | UHR | VHR |
The SAR Antenna Subsystem (SAS) is one of the CSG elements that compound the majority of technology and design innovations characterizing the CSG mission.
Development Status
• June 2018: The M-CDR (Mission-Critical Design Review) of the CSG mission has been completed in November 2017. The improved quality of the imaging service is among the foremost characteristics of CSG, providing the End Users with new/enhanced capabilities in terms of higher number of images and increased image quality (i.e. larger swath, and finer resolution) with respect to COSMO-SkyMed (first generation) spacecrafts currently in operation, along with additional capabilities (e.g. full polarimetric SAR acquisition mode, Theater acquisition, DI2S acquisition, Last minute planning, and Very Urgent Services). The main improvements for what concern the CSG spacecraft are: 10)
- Enhanced EPS (Electrical Power Subsystem) for an increased peak power required by the payload and longer operational life.
- Evolved AVS (Avionis Subsystem) which includes CMG introduction, STT enhancements, On board computer evolution, GPS evolution and Ring Laser Gyros introduction.
- Enhanced PRP with increased tank capacity.
- Enhanced TT&C with increased data rates.
- Enhanced SAR Payload with dual polarization on receive and interleave modes at PRI level (DI2S and Quad-Pol).
- Enhanced PDHT (Payload Data Handling and Transmission) with increased on-board memory and downlink data rate.
Support modes | Polarization | Access region (º) | Swath | Resolution | NESZ (dB) | Scientific geolocation (m) |
Spotlight 2A | Single/Dual | 20-25 | 3.1 x 7.3 | 0.35 x 0.55 | -23.5 |
|
Spotlight 2B | Single/Dual | 20-60 | 10 x 10 | 0.63 x 0.63 | -20 | 1.25 (90% CE) |
Spotlight 2C | Single/Dual | 20-25 |
|
| -22 |
|
Stripmap | Single/Dual | 20-50 | 40 x 40 | 3 x 3 | -22 | 2 (90% CE) |
Ping-Pong | Dual/Quad | 20-50 | 40 x 40 | 12 x 5 | -24 | 10 (3σ) |
Stripmap Quadpol | Quad | 20-45 | 40 x 15 | 3 x 3 | -25 | 2 (90% CE) |
ScanSAR 1 | Single/Dual | 20-60 | 100 x 100 | 20 x 4 | -22 | 10 (3σ) |
ScanSAR 2 | Single/Dual | 20-50 | 200 x 200 | 40 x 6 | -22 | 10 (3σ) |
- Geolocation accuracy: CSG features different levels of geolocation accuracy, depending on the availability of orbital data of in-creased accuracy:
a) “fast delivery” accuracy: 25 m (3σ). It is based on the orbital data downloaded by the satellite inside the product raw data stream
b) “standard” accuracy: 3.75 m 90% Circular Error (CE) for Stripmap and Spotlight products and 12 m (3σ) for Ping-Pong and ScanSAR modes. It is based on filtered orbits, provided several times per day by the Flight Dynamics Subsystems
c) “scientific” accuracy (Table 2), established thanks to orbits “reconstructed” on the basis of the data provided by the COSMO-SkyMed GPS Fiducial Network. This level of accuracy will be available to non-governmental users one orbital cycle (16 days) after the product acquisition epoch.
• September 28, 2017: TAS (Thales Alenia Space) and Arianespace have signed a launch contract for two COSMO-SkyMed Second-Generation (CSG) satellites manufactured for the Italian Space Agency (ASI) and the Italian Ministry of Defense. 11)
- The signing ceremony was attended by French President Emmanuel Macron and Paolo Gentiloni, the President of the Council of Ministers of the Italian Republic, at the Prefecture du Rhône, in Lyon, France, on the occasion of the 34th French-Italian summit.
- In addition, Arianespace and ELV/AVIO announced the signature of a contract for Arianespace to order 10 Vega and Vega C launchers from the Italian manufacturer. These 10 additional Vega and Vega C launchers will enter service starting in 2019, from the Guiana Space Center, Kourou.
• June 2016: The whole system first + second generation, namely CSG, has been designed and implemented in accordance with the IEM (Interoperability, Expandability and Multisensor) requirements. Thanks to IEM capabilities CSG is not a mere evolution of the first generation, it is more than the simple “sum” of first and second generation constellations: it is a “system of systems” providing “new” capabilities coming from the synergic utilization of both constellations. 12)
- The first generation of the system (usually labeled CSK) has been in operations since 2007 and the second generation (called CSG) is being developed enhancing the Interoperability, Expandability and Multi-sensor characteristics. The first generation system has been deployed with two Italian User Ground Centers (one civilian, one military) and expanded to a partner User Ground Center (located in France), two Mobile Acquisition and Processing Stations, and several Distant Cells and Portable User Terminals.
• In 2014, the CSG program is in Phase C, a CDR (Critical Design Review is scheduled for Q1 2015 (Ref 3).
Spacecraft launch mass | ≤2230 kg |
Spacecraft design life | 7 years |
Operative autonomy | 24 hours |
Navigation and time reference | GPS receiver |
RF communications | - X-band downlink for payload data, data rate: 2 x 260 Mbit/s |
Onboard data storage capacity | 1530 Gbit at EOL |
Launch
Launch of CSG-1
The first satellite of the CSG constellation, CSG-1, was launched on 18 December 2019 (08: 54:20 UTC) with an Arianespace Soyuz vehicle from the Guiana Space Center in French Guiana. The Soyuz 2-1b (Soyuz ST-B) rocket used a Fregat upper stage. Flight VS23 was Arianespace’s third launch in 2019 using a medium-lift Soyuz, and the ninth overall this year across its full family of launchers – which also includes the heavy-lift Ariane 5 and lightweight Vega. 13) 14) 15) 16) 17) 18)
The first telemetry data sent by the CSG-1 satellite was acquired by the Telespazio's Fucino Space Center 26 minutes after the launch. In the coming days the LEOP phase (Launch and Early Orbit Phase) will be run, followed by the satellite being put through the IOT (In Orbit Test) and Operational Qualification phases.
Secondary payloads
• CHEOPS (CHaracterizing ExOPlanets Satellite) minisatellite of ESA with a mass of 300 kg. 19)
• EyeSat, a 3U CubeSat (5 kg) student satellite of the University Technological Institute (IUT) in Cachan, France and CNES. 20)
• ANGELS (ARGOS Néo on a Generic Economical and Light Satellite), a microsatellite (30 kg) of CNES and NEXEYA, an innovative industrial group active in the aerospace market.
• OPS-SAT, a 3U CubeSat (6 kg) of ESA/ESOC that will allow on-orbit testing and demonstration of experimental and innovative software that may be used in future ESA missions and programs.
Orbit: Circular sun-synchronous dawn-dusk orbit, nominal altitude = 619.6 km, inclination = 97.86º, period = 97.1 min, with LTAN (Local Time of Ascending Node) at 6:00 hours (dawn/dust orbit), 14.8125 rev./day (or 14 13/16). All spacecraft of the SAR constellation will be positioned in the same orbital plane.

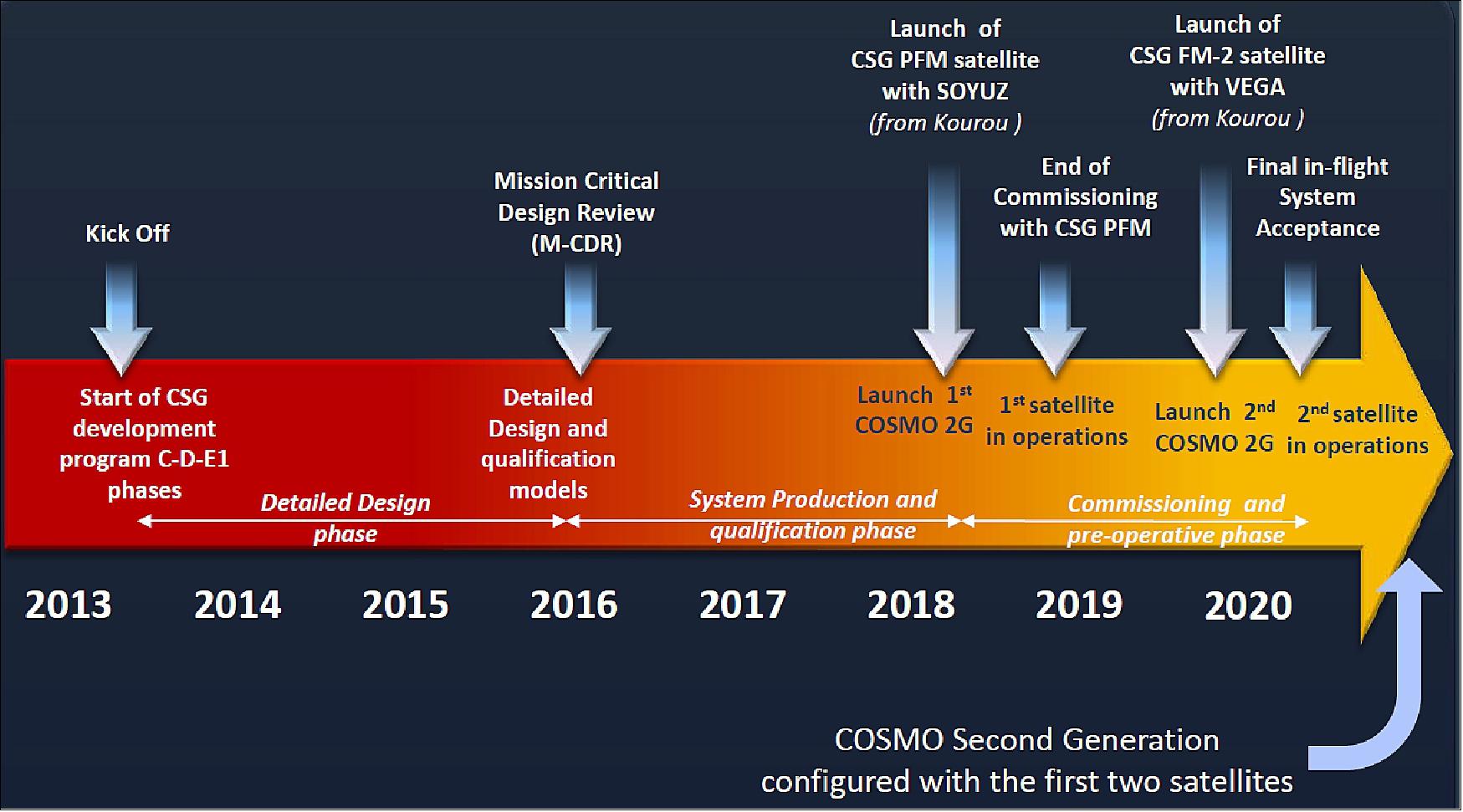
Launch of CSG-2
On January 31, 2022, a SpaceX Falcon 9 placed an Italian radar mapping satellite into orbit after four days of delays caused by range and weather problems. 21)
The Falcon 9 lifted off from Space Launch Complex 40 at Cape Canaveral Space Force Station in Florida at 6:11 p.m. EST (23:11 UTC). The rocket’s upper stage deployed its payload, the Cosmo-SkyMed Second Generation (CSG- 2) satellite for the Italian space agency ASI and the Italian military, an hour after liftoff.
The rocket’s first stage made a landing back at Landing Zone 1 at Cape Canaveral eight minutes after liftoff. The booster was originally built as a side booster for the triple-core Falcon Heavy rocket and flew on the second and third Falcon Heavy missions in 2019. This was the first launch where a Falcon Heavy side booster had been converted into a Falcon 9 booster.
The launch was originally scheduled for Jan. 27 but was scrubbed by poor weather, which also postponed launch attempts the next two days. Weather cooperated for a fourth attempt Jan. 30, but a cruise ship sailed into restricted waters off the Florida coast and could not move out of the way in time for the launch.
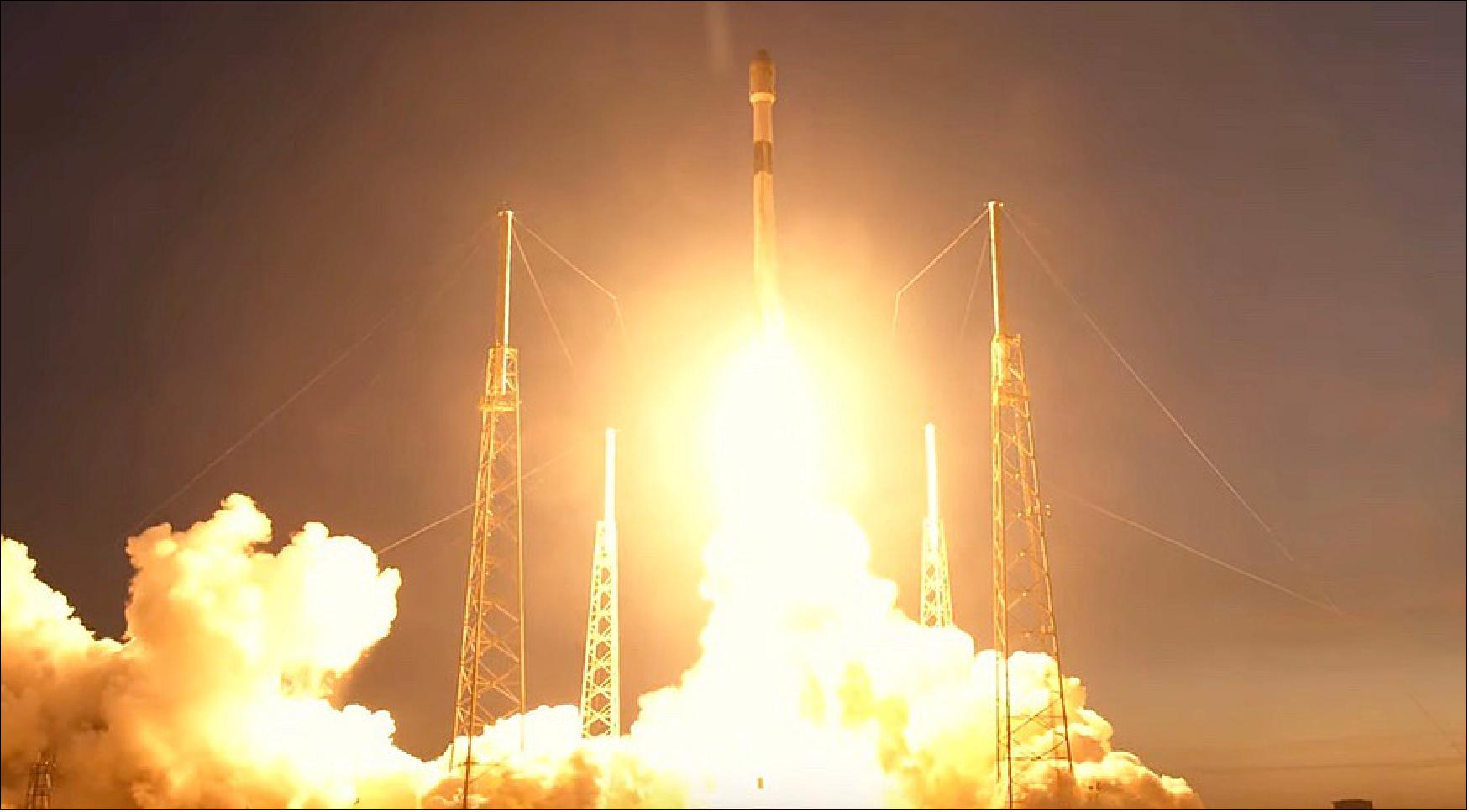
The 2,200 kg satellite, built by Thales Alenia Space, is the second in a fleet of four satellites intended to replace the original Cosmo-SkyMed satellites launched between 2007 and 2010. The second-generation satellites provide X-band synthetic aperture radar imagery, like the first-generation satellites, but can also collect images of two regions hundreds of kilometers apart, simultaneously.
CSG-2 was originally slated to launch on a Vega C. However, with the first launch of that vehicle delayed until at least May, ASI elected last fall to move the launch to a Falcon 9 in the hopes of launching the spacecraft by the end of the year, a decision that raised eyebrows among some in the in the European space industry.
“Since Arianespace backlog was already full on Soyuz and Ariane systems in 2021, it was not possible to have a European back-up solution compliant with the CSG-2 schedule,” ASI said in a statement last fall justifying its decision to acquire a launch from SpaceX. “In line with its long-lasting support ensured to the European launch industry, ASI confirmed its trust in Arianespace and Vega C capabilities by contracting the launch of the CSG-3 satellite, planned for 2024.”
Mission Status
• April 27, 2021: COSMO-SkyMed Second Generation (CSG) is now part of ESA's Third Party Missions Programme, in which ESA has an agreement with ASI to distribute data products from the mission. COSMO-SkyMed Second Generation consists of two enhanced SAR satellites: CSG-1, launched in 2019 and CSG-2, to be launched in 2021. 22)
- CSG’s objective is to provide data on a global scale to support a variety of applications among which include risk management, cartography, forest and environment protection, natural resources exploration, land management, defence and security, maritime surveillance, food and agriculture management.
- The COSMO-SkyMed Second Generation data are available for scientific research and application development (both archived and new acquisitions), following the approval of a project proposal.
- ESA will support as many high-quality and innovative projects as possible within the quota limit available, therefore only a limited amount of products can be made available to each project. Details about the data provision, data access conditions and quota assignment procedure are described in the CSG Terms of Applicability.
Sensor Complement
CSG-SAR (COSMO-SkyMed Second Generation Synthetic Aperture Radar)
The new SAR instrument represents the true enabling core of the CSG system. The top goal is to enhance the quality of the image products preserving the observation flexibility. Indeed, the CSG-SAR imaging capabilities at different resolution and sizes will include narrow field images taken at very high resolution (i.e. spotlight mode) devoted to intelligence and defense applications, as well as a large suite of civilian applications supported by narrow field images taken at fine resolution and simultaneous double polarization (i.e. Spotlight 2 mode), throughout wide field images (i.e. stripmap) taken at mid resolution (i.e. 3 m x 3 m) and double polarization, or wide swath width images (ScanSAR) taken at low resolution (> 4 m) and double polarization as well.
The new/improved functions are reflected in SAR sensor design and architectural innovations both in SAR antenna and SAR electronics. The CSG-SAR antenna modular design consists of ten identical “macro tiles”, with a high degree of electronics integration and density. Each macro-tile will be capable to manage signals at a very large pulse bandwidth and to simultaneously receive radar signals in H and V polarizations, through two independent channels. Each macro-tile contains a double number of TRMs (Transmit Receive Modules) with respect to CSK, on a surface of the same dimensions, along with service and control units:
• Macro-tile TRMs: The enabling technologies of the new TRM design are based on sophisticated RF MMIC (Monolithic Microwave Integrated Circuit) microelectronics, realized in GaAs space qualified technology and suitable for very high RF output power and efficiency that largely exceed the levels provided today.
• Marco-tile Service Units: The service units, such as the digital TCU (Tile Control Unit), the BFN (Beam Forming Network), the TDL (True Delay Line) are necessary to ensure the beam collimation over the whole operative frequency band, the TPSUs (Tile Power Supply Unit) which are all designed in order to exploit the augmented performance in terms of RF transmission and dissipated power (+40% with respect to the current CSK system).
On the SAR electronics side, the innovations of interest are:
• DEE (Digital Electronics Equipment) Tx Section: As regards the radar waveform generator, the CSG design is based on the DDS (Direct Digital Synthesis) at intermediate frequency of the Tx signal, allowing a significant improvement of the performance provided by the transmitter (spectral purity of the generated waveform and augmented flexibility). Furthermore, the novel generator implements a waveform pre-warping, in order to compensate for the distortions due to the analog electronics and to the DAC. The employed compensation functions are completely reprogrammable and can be adapted to the bandwidth required for the selected measurement mode.
• DEE Rx Section: On the receiver side, the requirement of managing a bandwidth improved with respect to the first generation, joined with the need of improving the figures of merit relevant to the produced images, has led to the implementation of I/Q demodulation in the digital domain, in order to avoid the distortions and unbalances in amplitude and phase between the I and Q channels typical of an analog device. The approach implies the need for the signal to be sampled directly at the intermediate frequency (ADC sampling at frequency equal to 1.4 GHz), and therefore requires for higher processing speed. Furthermore, an improvement in the precision of the SAR data digitization allows the SAR sensor to manage a wider dynamic range of received signals. To reduce the data volume produced on-board, the novel digital receiver implements filtering and decimation (programmable decimation factors) through digital filters, while a further data reduction is achieved through the BAQ (Block Adaptive Quantizer). This approach allows minimizing the number of samples which are transmitted towards the SAR-PDHT interface (in any case four times bigger than CSK).
RFE (Radio Frequency Equipment): The SAR central electronics new design also involves its RFE capable to manage a considerably wider instantaneous bandwidth, to guarantee a tight control of the amplitude and phase errors in order to preserve the figures of merit of the impulse response function, and to generate all the reference signals required for the sensor timing. Key elements of the RF element are the newly designed wide-band receiver and wide-band transmitter, a double receiving channel for simultaneous double polarization, ensuring amplitude and phase matching between receiving channels, and the generation of reference signals at high stability.
The CSG-SAR is a multi-mode instrument, conceived to provide a wide range of performance through different implementations (measurement modes) of the three major SAR acquisition techniques (Stripmap, Spotlight and ScanSAR). 23) 24)
On the basis of a different management of the polarizations in Tx and Rx, three variants of the Stripmap technique are provided: Stripmap, Pingpong and Quadpol.
- Stripmap is a mode at medium resolution conceived for supporting products at 40 km of swath width, in either single (HH or HV or VV or VH) or dual polarization (HH&HV or VV&VH).
- Pingpong is a medium resolution mode which alternates different polarizations on a burst basis. As for the CSK-SAR, the instrument can operate in interleaved dual polarization (HH+VV): furthermore the CSG-SAR is also able to switch the Tx polarization on a burst basis and to receive simultaneously both horizontal and vertical polarizations (HH&HV+VV&VH).
- Quadpol is an experimental mode, implemented by doubling the PRF of the instrument and alternating the Tx polarization on PRI basis while receiving horizontal and vertical polarizations simultaneously (HH & HV & VV & VH).
In continuity with CSK, two ScanSAR modes are provided by the CSG-SAR. Moreover CSG offers also the capability of performing the ScanSAR acquisitions in dual polarization. ScanSAR-1 is a mode designed to achieve products with a swath of 100 km, in either single or dual polarization. ScanSAR-2 is a mode which assures products at 200 km of swath width, in either single or dual polarization.
The Spotlight technique is employed to provide modes at high and very high resolution. Four implementations of this technique have been envisaged in order to achieve various performance figures in terms of resolution, swath and polarization.
1) Spotlight-1A is a very high resolution mode at single polarization.
2) Spotlight-1B is a very high resolution mode at dual polarization.
3) Spotlight-2A is a high resolution mode at single polarization.
4) Spotlight-2B is a high resolution mode at dual polarization.
Mode category | Acquisition technique | Resolution (rg x az) | Swath (rg x az) | Polarization | User type |
|
|
|
|
| Defense |
|
|
| |||
0.8 m x 0.8 m | 10 km x 10 km | Single polarization |
| ||
1.0 m x 1.0 m | 10 km x 10 km | Double polarization | |||
|
| 3.0 m x 3.0 m | 40 km x 2500 km | Double polarization | |
5.0 m x 20 m | 30 km x 2500 km | Burst double polarization | |||
3.0 m x 3.0 m | 15 km x 2500 km | Quadruple polarization | |||
ScanSAR | 4.0 m x 20 m | 100 km x 2500 km | Double polarization | ||
6.0 m x 20 m | 200 km x 2500 km | Double polarization |
Imaging mode performance upgrade: 25)
The experience gained with the operational exploitation of the first generation system, shows that users have various needs according to their domain of belonging (defence, civilian institutional, civilian scientific and commercial). These needs have been continuously reviewed during the development of the system (phase A, B and C) and led to the following achievements:
• Very High resolution data: This enhancement represents the more evident and intuitive innovation of CSG with respect to CSK. CSG not only inherits the operative modes of the former CSK sensor improving their performances, but also introduces a number of new modes to provide a wider set of products in terms of coverage and geometric resolution. The resolution improvement will be achieved exploiting:
- The whole sensor available bandwidth for each incidence angle (thus marking a significant difference with respect to CSK that provides a constant geometric resolution for different incidence angles.
- An improved azimuth beam steering capability. A trade-off dealing with resolution, swath, NESZ and ambiguity to signal ratio, is on going and will be carried on to optimize the achievable resolution and image quality. Performances will be tailored according to the dual use requirements and overall operational concept, anyway CSG products will have a resolution that is more than twice improved with respect to the CSK one. Moreover the users will have the possibility to request the inherent resolution (resulting in a product with a rectangular pixel) or choosing from a series of levels of multi-looking in order to increase the radiometric resolution of the final product.
• High density of acquisitions: CSG will increase, with respect to CSK, the number of images acquired on the same area of interest. To this aim:
- Satellite agility, both at payload and platform level, will allow to mitigate constraints coming from the temporal overlapping among different acquisitions. Satellite agility is achieved through the introduction of a CMG (Control Moment Gyro) in CSG, so enabling a new experimental feature called “theatre acquisition”. The “theatre acquisition” relies on the acquisition of squinted spotlight images, the fast and precise maneuvering of the platform to change the payload pointing and the precise focusing of high squint spotlight acquisitions.
- The time gap between two following acquisitions will be optimized for specific sequences of imaging modes and acquisition geometries.
- Other experimental and innovative techniques, such as DI2S (Discrete Stepped Strip) Multi-Swath, are under investigation to allow the simultaneous acquisition of two targets.
Exploiting these enhanced experimental capabilities, CSG will be able to overcome situation like that one depicted in Figure 8 (top left), where two targets of interests (T2 and T3) cannot be both observed in the same overpass of the area (being the cross track separation of the two targets not compatible with the swath of the required product or being compatible only with wider swath modes at the expenses of geometric resolution). Figure 8 (top right) shows how the four targets can be imaged in a single “theatre acquisition”, where the imaging of all the targets (including T1 and T4) is performed while the satellite changes its attitude (pitch maneuvering); Figure 8 (bottom) shows the alternative approach based on the DI2S (Discrete Stepped Strip) Multi-swath, still under evaluation, that allows imaging simultaneously T2 and T3 preserving the nominal (i.e. zero Doppler) imaging condition for T1 and T4.
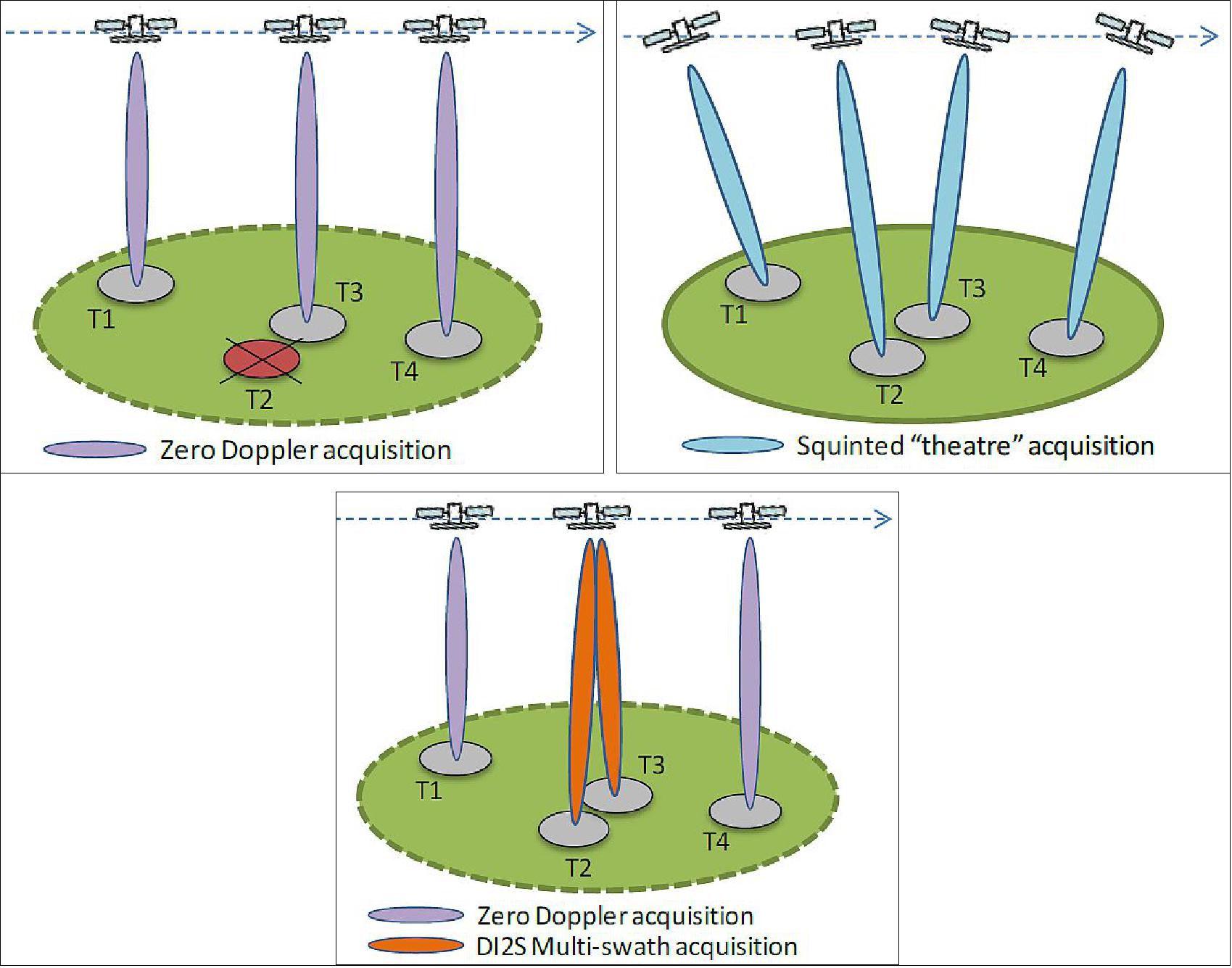
• Larger Spotlight swath width at very high resolution: CSG will implement specific experimental imaging modes in order to increase the observed area at higher resolution. In this regard, contiguous spotlight images would be acquired, exploiting “theatre acquisition” mode, thus increasing the nominal spotlight swath preserving the geometric resolution. Figure 9 shows one of the available geometric configurations where the size of the imaged area is doubled both in azimuth and range directions; other configurations foresee a triple swath only in one direction (range or azimuth).
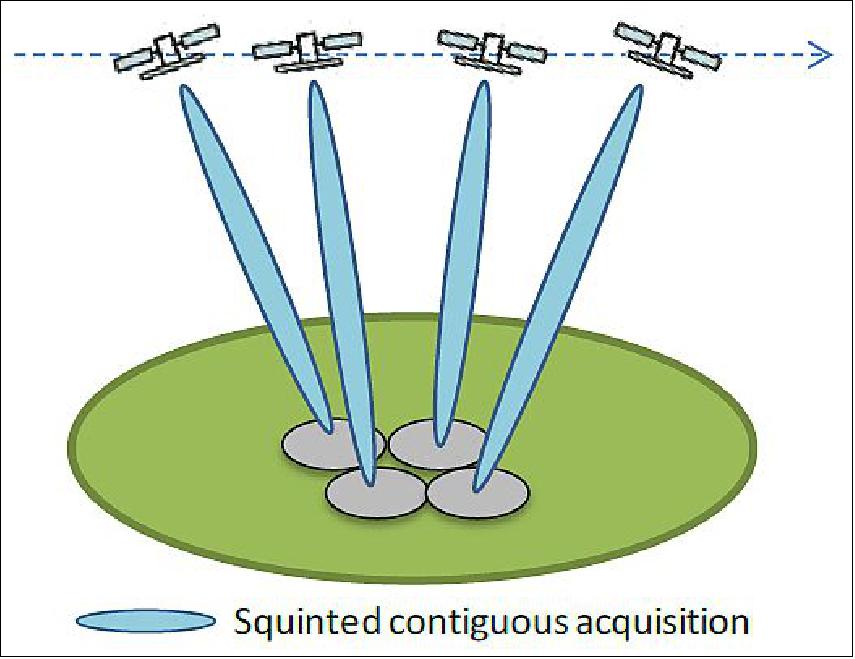
• Polarimetric sensing: CSG will provide user with both Dual pol and Quad-Pol data. While the Quad-Pol will be a specific imaging mode, the Dual-Pol feature will be available for all the standard imaging modes. In this regard:
- The antenna design has been updated and encompasses two separate receiving channels
- The Payload Data Handling and Transmission performance has been improved to manage the significant increase of the overall data volume. CSG system will be therefore one of the first systems providing this versatile polarimetric capability in X-band.
• Repetitiveness of the acquisitions: CSG will better support the need for periodic and coherent imaging of specific area of interest (e.g. MapItaly program to periodically acquire a full coverage of the Italian territory). To this aim, the major area of improvement was identified in the planning of acquisitions. The ground segment has been updated with the following features: 26)
- The introduction of a new planning capability enabling the pre-allocation of orbit resources for mid-term planning.
- Through the introduction of an advanced SAR parameter programming function that defines the SAR acquisition parameters taking into account the objective to preserve along time, for each observed area, the same sensing parameters [e.g. swath dimension, SAR PRF (Pulse Repetition Frequency)].
• Improved Background acquisition capability: CSG will manage periodic acquisitions on target areas of interest by exploiting long term planning functions. In this regards, the areas for improvement were identified in the automatic complete filling of the mission plan after the completion of the priority and user driven planning. Moreover, CSG will perform, as a low priority task, a large area coverage (i.e. world-wide) exploiting the daily imaging resources unused after the on-demand planning of the acquisitions. While for the priority requests a rank-based planning strategy will be used, this background acquisitions will be planned in order to optimize and fully exploit all the imaging resources of the system.
Flexibility and responsiveness
In the design of CSG system, priority has been given to the implementation of those features that allow to increase the ability of the system to satisfy the needs of the various user classes (defence, institutional, scientific, commercial) and their simultaneous requests and priorities. This objective can be pursued through the minimization, as much as possible, of requests rejections due to direct conflicts or system resources depletion. In this regard, the following area of improvement were identified and addressed:
• Flexibility in the exploitation of the imaging resources. The planning strategy considers the imaging resources (Satellite’s Energy Resources, On-board Memory Resources, Ground Segment Resources) as dynamic variables, which vary as function of resources consumption and restoration. Therefore, each acquisition request will be considered suitable or not for the specific acquisition plan, according to the actual availability of system resources.
• Valorization of commonalities among different users. In this regard, the improvements involve the following features:
- The sharing of common programming requests among different users by means of the cooperative environment available within the ground segment (this feature is available in both civilian and defence domain).
- The reduction of time needed for the maneuvers (by means of CMG), that significantly decreases the satellite unavailability for maneuvering.
- Request order refinement to exploit the agility of the system increasing the density of acquisitions that can be served on an area of interest.
• Priority allocation and management: to manage the unrecoverable conflicts among requests of different users, belonging to national and international Partners and to different classes (i.e. defence and civilian), CSG will:
- Encompasses a fair intra-class request harmonization algorithm
- Make efficient the inter-class requests list harmonization by serializing the defence and civilian planning process.
• User satisfaction is also closely linked to the timely provision of data. Improvements in this area were also investigated and, at the present design stage, the following updates are foreseen:
- Very Urgent as a service: CSG will provide, in continuity with the first generation of COSMO-SkyMed, the possibility to define a request outside the nominal mission chronology, being this asynchronous request to be served as fast as possible. The management of this VU request will be handled by the system as a specific service with maximum priority, without any need to switch the overall system to a dedicated asynchronous chronology, as was for CSK. This implies the capability of the system to manage simultaneously a synchronous and an asynchronous planning cycle.
- Pre-booking and last minute planning refinement: This function allows users to preliminary define an area of interest and then refine their acquisition order as late as possible. In some respect this will allow the final user to “book” an acquisition time window and to define the actual image acquisition just before the last S-band uplink contact of the satellite preceding the data take’s execution, thus improving dramatically the system response time perceived by the end user. This function is enabled by the asynchronous programming that had been made completely compatible with the main synchronous one.
- Near Real Time data delivery: CSG will allow the user, when the acquisition follows into his X-band receiving station visibility, to force the direct download of the data to his receiving station. To this aim, the planning function was revised allowing an efficient and optimized management of the downlink opportunities decoupled from the main planning of the data take opportunities and its associated priorities.
Interoperability, expandability and multi-mission/multi-sensor
CSG has been developed, since its early stage, as an answer to national needs and requirements but also as an opportunity to foster international cooperation and the exchange/integration of capability among different systems.
• Multi-mission/multi-sensor is the capability provided by CSG to task and manage several sensors/missions in an integrated ground segment environment. This allows:
- Minimizing the operational effort though the reduction and optimization of logistics and operational tasks/personnel
- Optimizing the overall image data collection process through the effective and automatic coordination of the different sensors/missions tasking (e.g. managing multi-mission multi-sensor programming order for optical and/or SAR products). CSG provides these multi-mission features through the development of a specific module of the UGS (User Ground System), called Multi-Sensor Interfacing UGS (MSI-UGS), representing the unique front end of the final user.
• Interoperability with external system has been studied since the beginning of the program to achieve multi-mission/sensor capabilities. From a defence perspective, this was to be realized in MUSIS (Multinational Space-based Imaging System), being CSG one of its components. At this stage, both CSG and the MUSIS program evolved; CSG interoperability enabling features and capabilities where consolidated and they are now considered in the design of the MUSIS Common Interoperability Layer in order to allow the CSG UGS to provide the Italian user with services from the French Composant Spatiale Optique system (and vice versa for the French users). For the civilian side, ASI has in place an international cooperation with the Argentinean Space Agency (CONAE) that will result in a common system SIASGE (Sistema Italo Argentino de Satélites para la Gestión de Emergencias - Italian/Argentinian satellite system for emergency management) based on the integration between COSMO-SkyMed and the L- band SAOCOM (SAR Observation & Communications Satellite) system.
• Expandability of the CSG system is intended as the ability to serve a Partner or an external user trough an expansion of the CSG ground segment architecture. This expansion is natively conceived, as well as the main system, to be multi-mission/multi-sensor, therefore able to provide all the interoperable service to the CSG final users.
• The CSG system will provide operative continuity on dual-use services to CSK, with better performances through the significant upgrade of its operational and exploitation concepts, enabled by key technology advances and an innovative system design. These improvements support the main purpose of the Italian Space Agency and Ministry of Defence to broaden the range of SAR imagery applications for a wider End Users’ community, while multiplying efficiency and versatility of the provided services (Ref. 25).
Standard Operative modes: The CSG System is able to provide, as standard SAR instrument operative modes, narrow and wide field acquisition modes (i.e. Spotlight, Stripmap, ScanSAR), exhibiting both fine on-ground resolutions and wide swath, introducing a double polarization information as well. The current CSG standard operative modes are: 27)
- Spotlight-1A
- Spotlight-1B
- Spotlight-2A
- Spotlight-2B
- Spotlight-2C
- Stripmap
- Pingpong
- Quadpol
- ScanSAR-1
- ScanSAR-2
With the aim to better exploit the system potential, without introducing operational constraints, the main enhancement with respect to the first generation is in particular for the spotlight modes with three different approaches achieved:
- Optimization of resolution performance (Spotlight-2A)
- Optimization of swath performance (Spotlight-2B)
- Optimization of satellite resources (Spotlight-2C) to increase number of images feasible (the performance has to be finalized).
The performance, reported in Table 5 that will be frozen at M-CDR (Mission-Critical Design Review); the new operative modes will allow the CSG system to be competitive in the current international Earth observation system market.
Mode | Resolution: Azimuth x Range (m x m) | Swath Azimuth x Range (km x km) |
Spotlight-2A | 0.35 x 0.48/0.55 (depending on incidence angle) | 3 x 8 |
Spotlight-2B | 0.63 x 0.63 | 10 x 10 |
Stripmap | 3 x 3 | 40 x 10 |
Pingpong | 12 x 5 | 30 x 30 |
Quadpol | 3 x 3 | 40 x 16 |
ScanSAR-1 | 20 x 4 | 100 x 100 |
ScanSAR-2 | 40 x 6 | 200 x 200 |
Non-Standard/Experimental Operative modes
The non-standard/experimental operative modes in addition to increase the user portfolio contribute to strengthen the CSG system even enhancing the performance beyond the actual limits. Challenging but very interesting experimental modes are introduced exploiting some engineering and technological improvements:
- DI2S Spotlight-1 (DIscrete Stepped Strip): MS-OR (Multi-Swath Optimized Resolution)
- DI2S Spotlight-2 (DIscrete Stepped Strip): MS-OS (Multi-Swath Optimized Swath)
- Spotlight scattered on theater
- Spotlight adjacent on theater.
DI2S Multi-Swath allows to achieve a couple of images simultaneously acquired, increasing the number of acquirable images on a given area otherwise not feasible for time gap violation. In particular, the DI2S Multi-Swath Spotlight solution provides two images with the same azimuth position, two images shifted in azimuth, two images with same range shifted in azimuth (Figure 10). In particular, the two DI2S operative modes (MS-OR and MS-OS) allow to optimize the resolution or swath performance, respectively.
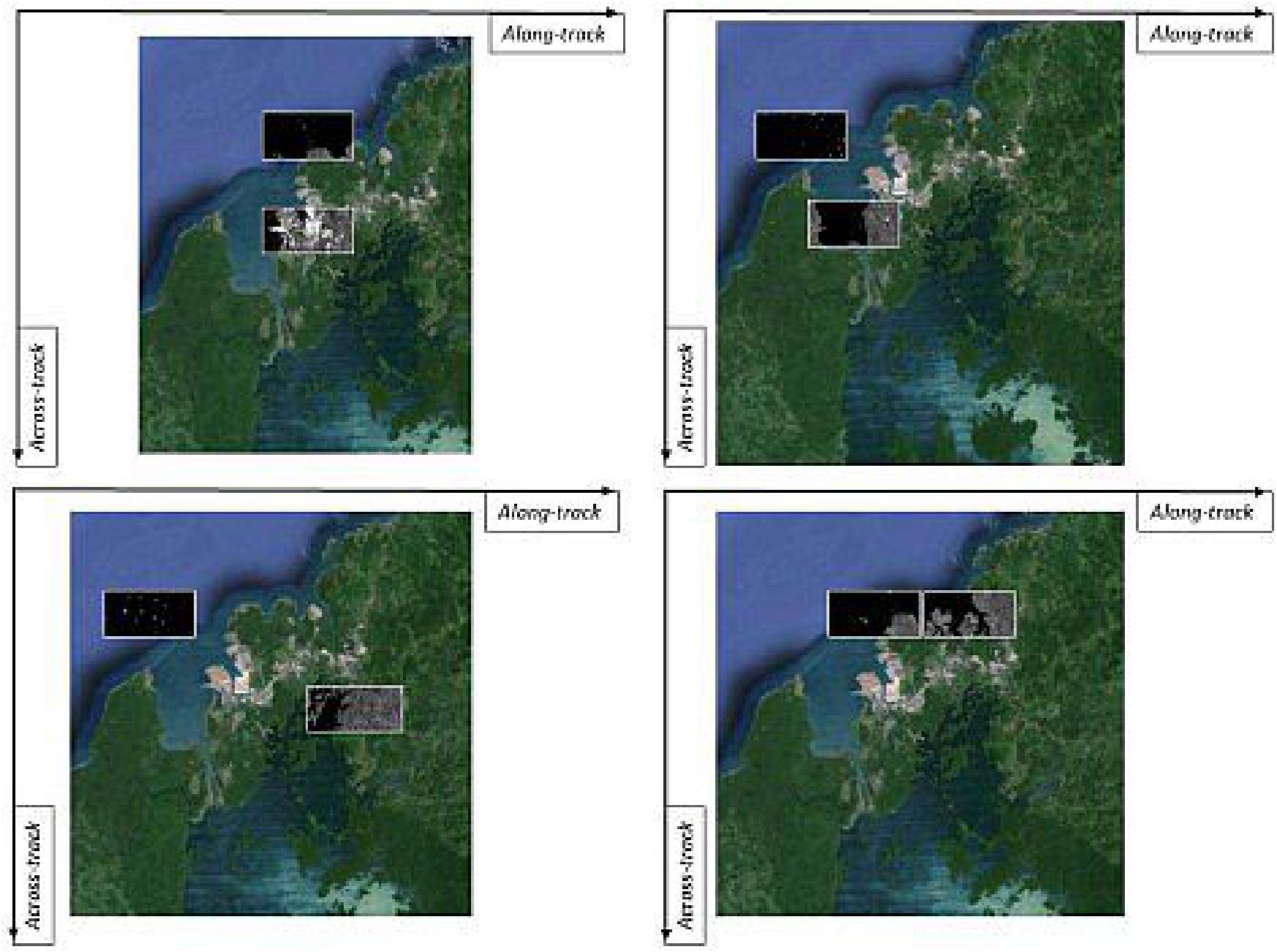
Squinted acquisitions on Theater Scenario can be used to serve more requests in a defined area, that otherwise could not be served because in conflict for time gap violation. The CSG System, by exhibiting a very high satellite agility performance, will be able to perform this functionality. The two CSG non-standard/experimental operative modes (scattered and adjacent) represent only the approach (scattered or adjacent) used by the satellite to acquire the images on the theatre area. The following samples in Figure11 provide an example of possible adjacent configurations achievable on a theater scenario by using the standard Spotlight operative mode.
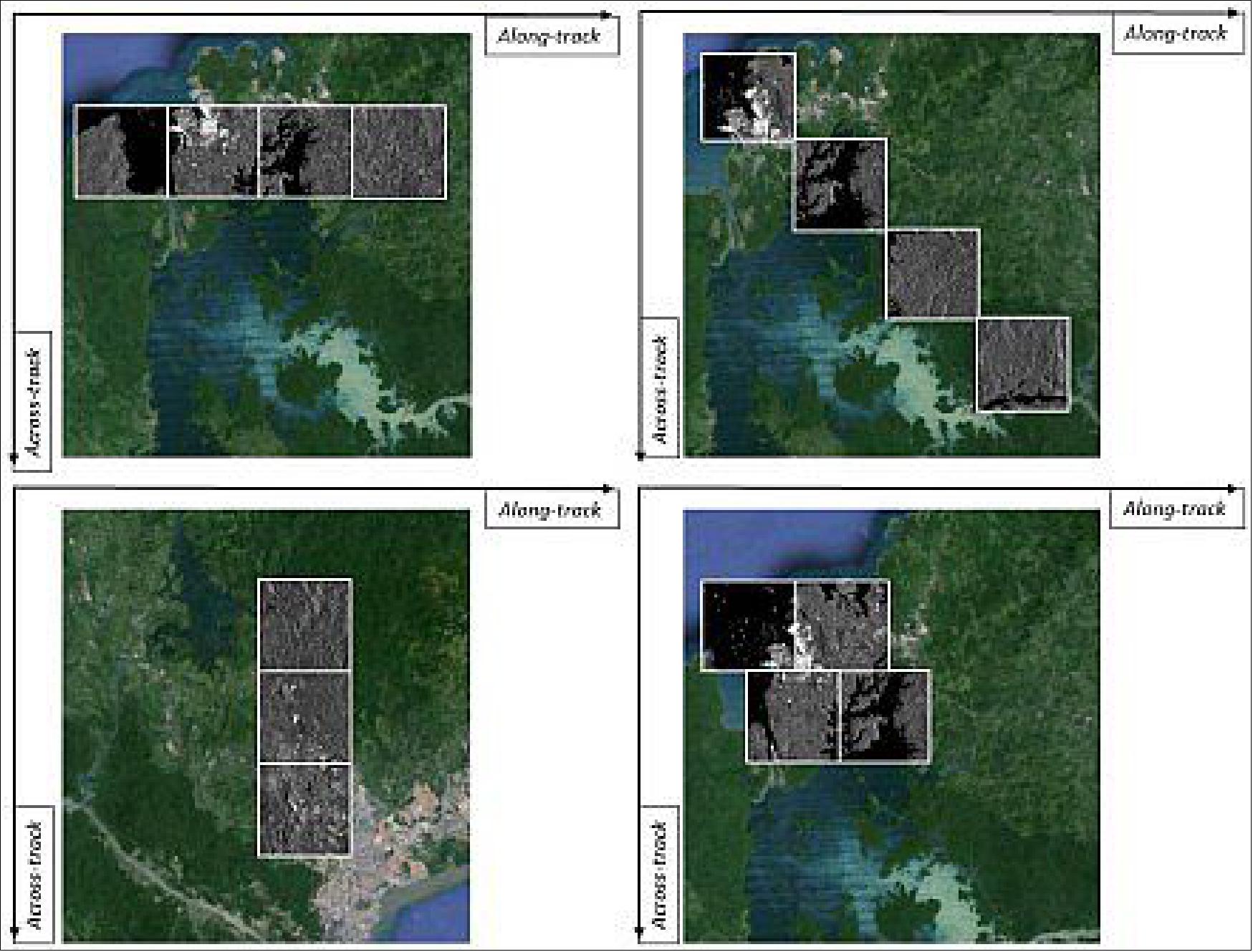
Instrument architecture
Figure 12 outlines a functional block diagram of the CSG-SAR. The functions implemented by the instrument are allocated within two major subsystems: SES (SAR Electronic Subsystem) and SAS (SAR Antenna Subsystem). 28)
The radar signal is generated at a proper IF (Intermediate Frequency) by the chirp generator and up-converted to the X-band. The up-converter includes also a frequency multiplier, which contributes to bring the center of the spectrum of the pulse up to the frequency used for its transmission and moreover stretches the bandwidth of the signal. Afterwards, the signal coming from the SES is distributed through the Tx BFN (Beam Forming Network) of the SAS to the HPAs (High Power Amplifiers) inside the TRMs of the antenna, and eventually the signal is radiated via the array radiators. The amplitude and phase controls of each TRM determine the key characteristics of the beam in terms of pointing, beamwidth and shape, while the TTDLs (True Time Delay Lines) are employed for avoiding beam pointing dispersion versus frequency. The SAS is conceived as a flexible item whose management is performed in synergism by the antenna itself, through the tile controllers, and by the Control Computer of the SES.
On reception, the echo signals captured by the array radiators are amplified by the LNAs (Low Noise Amplifiers) inside the TRMs and combined by two BFNs. The usage of two separate Rx BFNs is required in order to support simultaneous dual polarization on receive for those modes which employ this capability. Afterwards the received echoes are routed towards the Rx channels of the SES (for single polarization modes only one channel is used while for modes at dual and quad polarization, two Rx chains are employed simultaneously) to be amplified and down-converted to a proper IF before entering the digital section of the SES. In accordance with the approach successfully experienced in the CSK mission, the architecture of the SES is designed to enable the selection of two different receiving schemes: deramp-on-receive, for the Spotlight modes, and standard superheterodyne, for all the other modes. Finally, the received echoes are digitized, demodulated, filtered, decimated, compressed and formatted to be sent to the PDHT (Payload Data Handling and Transmission) system for their downlink.
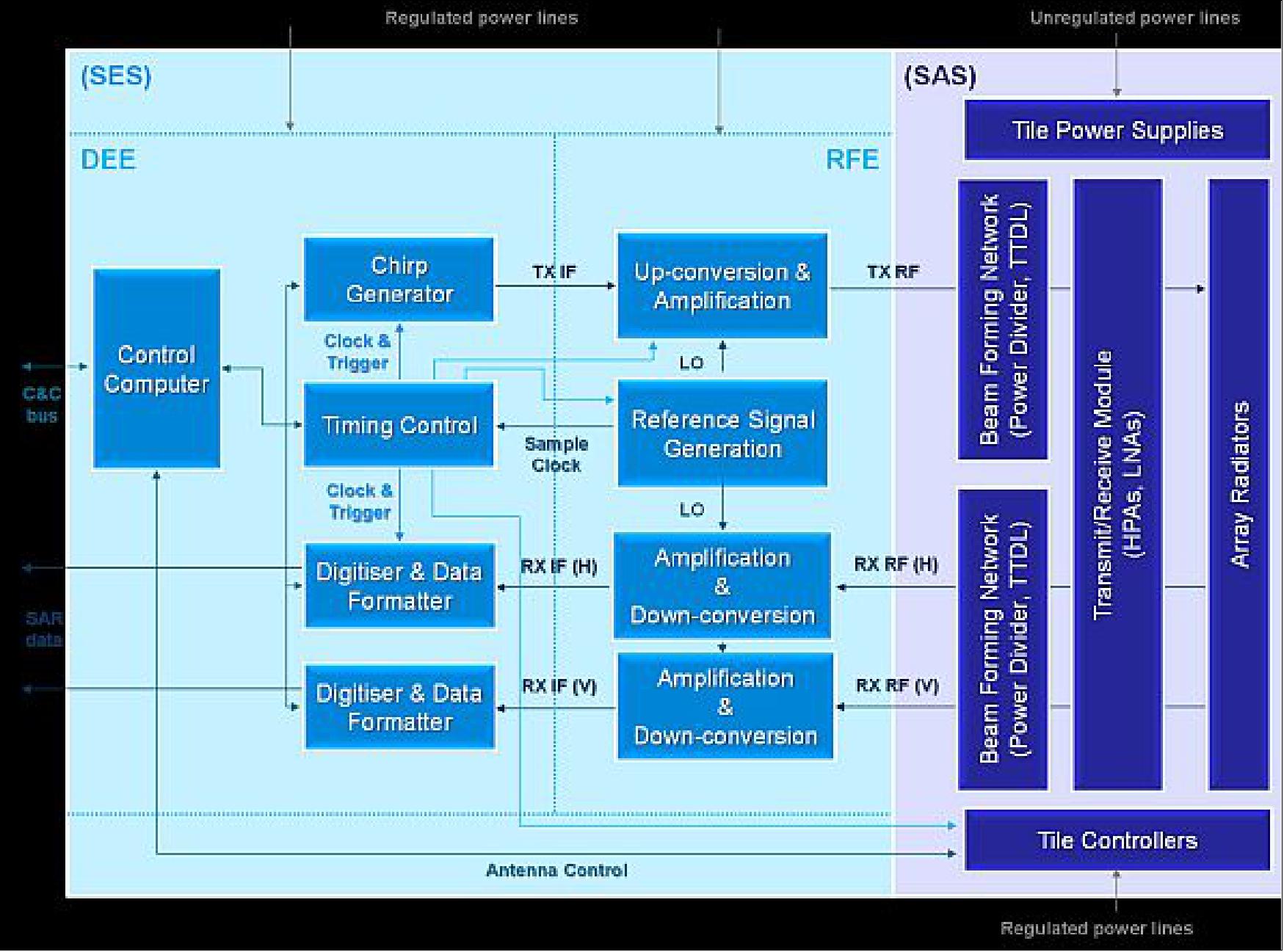
The functions described are required to support the primary tasks of the CSG-SAR, which are basically related to transmitting chirp signals and collecting their echoes. On the other hand, proper auxiliary features such as instrument control, timing, generation of reference frequencies and internal calibration are crucial in order to undertake these tasks in accordance with the required functionalities and performance.
Parameter | Value | Comment |
Center frequency | 9.6 GHz (adaptable to possible ITU regulation update) | Possible ITU regulation regarding bandwidth |
Bandwidth | Programmable | |
Polarization | Single, Dual, Quad | Selectable depending on measurement mode |
SAR antenna size | ~7.5 m2 |
|
Number of TRMs (Transmit Receive Modules) | 1280 @ H polarization and 1280 @ V polarization |
|
Pulse width | Programmable (5-100 µs) |
|
PRF (Pulse Repetition Frequency) | Programmable (500-7000 Hz) |
|
Data quantization | 10 bit |
|
BAQ data compression | 10:6, 10:5, 10:4, 10:3, 10:2, 10:1, 10:10 |
|
It is worth mentioning that the instrument design fully exploits the range of frequencies allocated by the current ITU normative for remote sensing applications in X-band, and is already compatible with possible future updating of this regulation.
SES (SAR Electronic Subsystem)
The functions implemented by the SES are allocated within the frame of two pieces of equipment: DEE (Digital Electronics Equipment) and RFE (Radio Frequency Equipment). 29)
The DEE contains all the elements needed to generate chirp pulses, to receive and process echo signals, and to generate timing signals. Furthermore the DEE plays the role of instrument controller, communicating with the spacecraft through the 1553 data bus, handling commands and control protocol, performing telemetry acquisition and commands propagation, generating and dispatching antenna parameters to SAS.
DEE implements the key functions of: Instrument control, signal generation and echo data acquisition. These functions are distributed between two different sections of the equipment: IC (Instrument Controller) and SC (Signal Conditioning).
The IC section comprises the following main functional blocks:
• CCU (Core Control Unit), which is devoted to communicate with the spacecraft through the 1553 data bus and to manage the command and control protocol of the SAR Instrument.
• Telemetry and Command I/F, which acquires the instrument telemetries and dispatches commands.
• MBC (Master Beam Controller), which is in charge of generating and distributing the antenna parameters to the SAS through the 1553 antenna bus.
• IC DC/DC, which generates all the supply voltages for the IC. The SC consists of the following modules:
- Mode Control Equipment, which is devoted to the generation of the instrument timing.
- Digital Chirp Generator, which synthesizes the chirp pulses to be transmitted.
- Echo Dig / BAQ / Formatter, which handles the acquisition of radar echoes and the delivery of the echo data to the PDHT (Payload Data Handling and Transmission subsystem).
- SC DC/DC, which generates all the supply voltages for the SC.
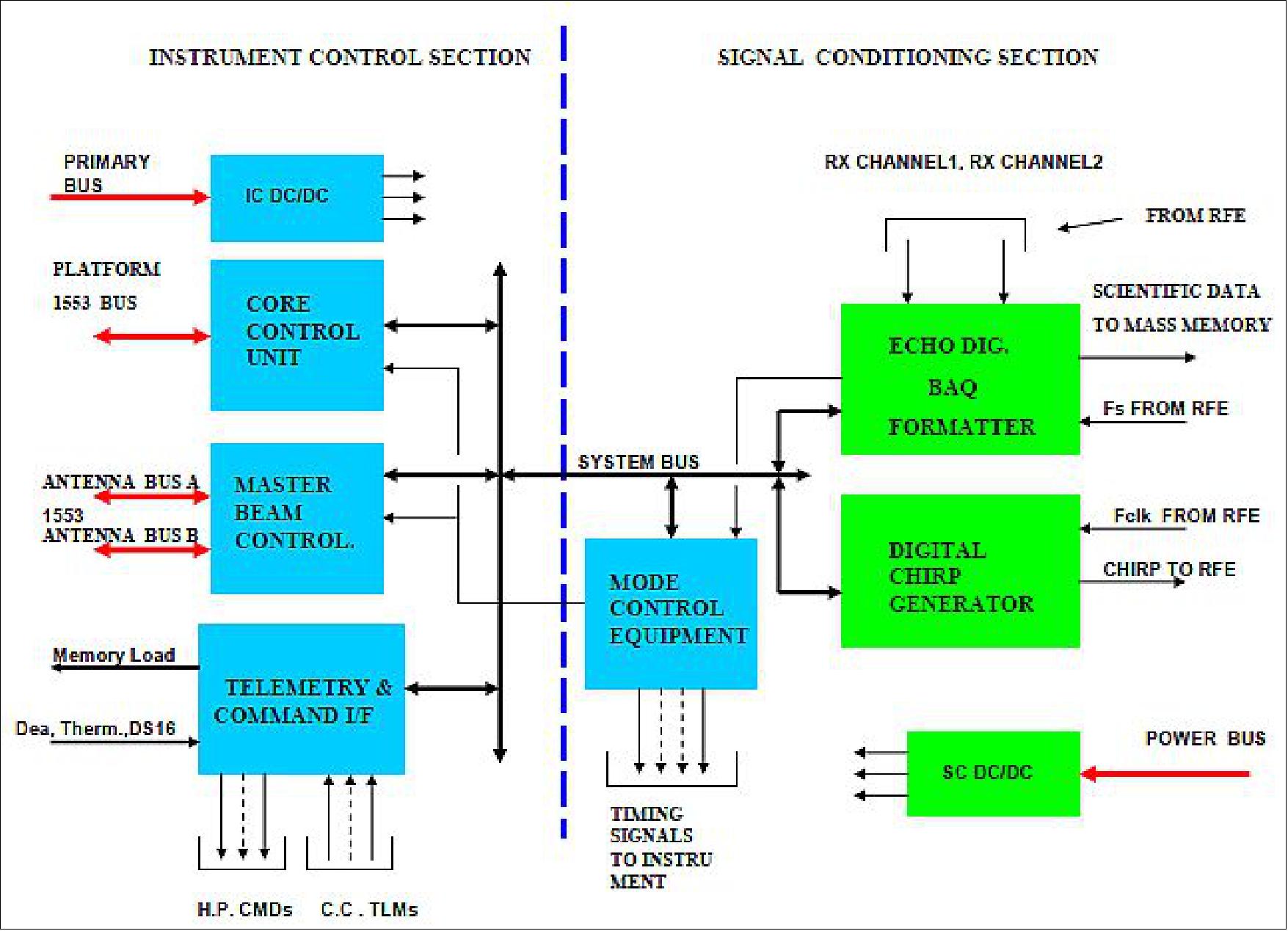
Two ASIC’s are used to implement the functions related to the generation of chirp pulses and to the digital processing of echoes, respectively. The first ASIC is a WFG (Waveform Generator) which implements direct digital synthesis functions for generating chirp pulses at programmable bandwidth, slope and duration. This solution allows avoiding the usage of an analog I&Q modulation, assuring a remarkable improvement of the spectral purity of Tx signals and augmenting the flexibility of the transmitter. Furthermore, this ASIC has the capability of compensating for amplitude and phase distortions of the hardware elements external to the DEE, while a dedicated FIR filter has the function of recovering the holding effect of the DAC. The WFG ASIC internal architecture relies on parallel chains, which are then multiplexed to be processed by a DAC at 12 bits. The requirement of synthesizing a bandwidth, which is more than double with respect to the CSK-SAR, and to achieve better performance figures push to increase the processing speed and justify the choice of adopting a 180 nm ATC ASIC.
The EDG (Echo Digit) is a complex ASIC which houses the functions of: I&Q demodulation, filtering and decimation, BAQ compression, CCSDS formatting, FIFO buffering, and interfacing with the PDHT. The requirement of managing an Rx bandwidth after deramping , which is more than threefold with respect CSK, joined with the need of improving the figures of merit relevant to the produced images, have driven to a complete innovation of the architecture of the I&Q demodulator, favoring the choice of a digital implementation of this device in order to avoid distortions and unbalances typical of an analog device. On the other hand, this solution involves higher processing speed. Furthermore, dealing with the section of data conditioning of this ASIC, it is worth mentioning that an improvement of a factor 4 in the precision of the SAR data digitization allows the SAR managing a wider dynamic range of received signals. Furthermore the DEG ASIC allows compressing the incoming I and Q data through a BAQ, which provides seven different compression ratios: 10:10, 10:6, 10:5, 10:4, 10:3, 10:2 and 10:1. Finally, the compressed signal is formatted and delivered to the PDHT in a CCSDS packet format through a Wizard Link interface. As for the WFG ASIC, in order to support the required processing speed, an implementation based on a 180 nm ATC ASIC has been chosen.
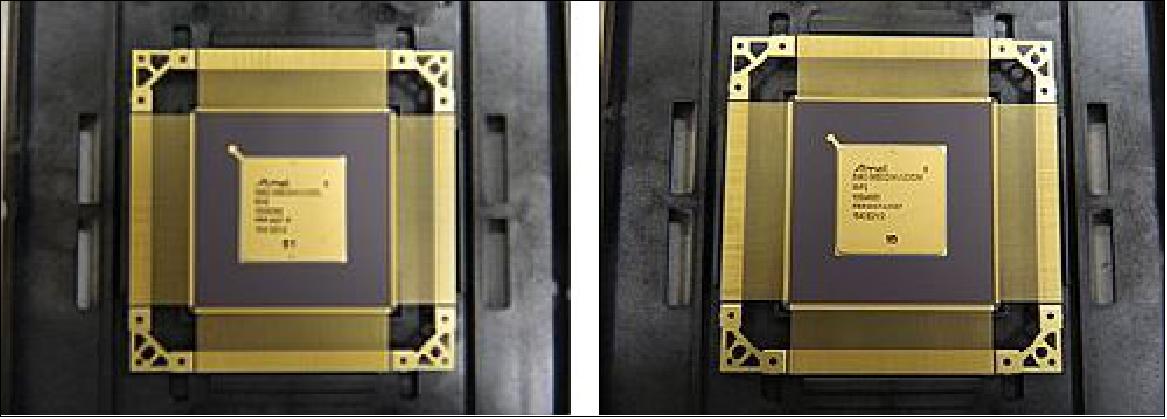
RFE (Radio Frequency Equipment): The RFE is charged of providing the functions of up-conversion & amplification, amplification & down-conversion, and reference signal generation. These functions, which are sketched in Figure 12, are implemented within three different sections of the RFE: Up-converter, Down-converter, and Frequency Generator.
The Up-converter assures the capability of transmitting signals whose maximum bandwidth is twice the one provided by the CSK-SAR instrument and handles deramping reference signals consistent with this bandwidth and with the required swath widths. This capability is founded on an architecture comprising an up-conversion mixer and a x 4 frequency multiplier.
The down-converter is based on a double conversion of frequency and comprises 2 parallel receiving chains for supporting the modes which require simultaneous dual polarization on receive. The first stage of down-conversion is implemented through a deramp-on-receive scheme for the Spotlight modes, whereas the Rx signals are converted by means of a standard superheterodyne receiver for all the other modes. The Rx gain can be adjusted upon command over a range of 30 dB in order to adapt the instrument dynamic on the basis of the expected echo level; furthermore, a dedicated attenuator is used to bring the level of the internal calibration signals within the receiver dynamic range.
In the Frequency Generator, all the reference frequency signals of the instrument are produced starting from an OCXO (Oven Controlled Crystal Oscillator) at 10 MHz which is used as a master reference.
Concerning the mechanical configuration of this equipment, it is worth noticing that in the CSK-SAR, the functions of the RFE were distributed among three different units, whilst in the CSG-SAR, thanks to the capabilities offered by state-of-the-art technologies, they are all enclosed within a single compact box.
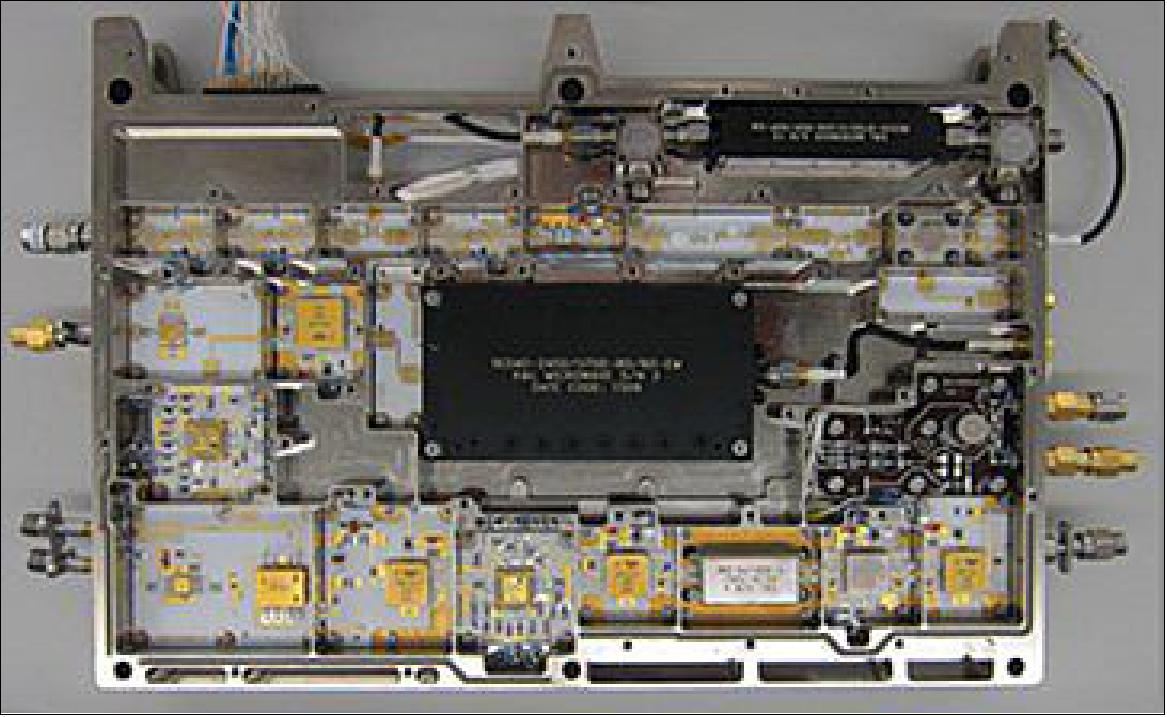
SAS (SAR Antenna Subsystem)
The CSG-SAR SAS is developed on the basis of the large experience gained on previous designs carried out at TAS (Thales Alenia Space) such as CSK-SAR and the KOMPSAT-5 SAR. On the other hand, the increased performance requirements in terms of bandwidth, radiated power and simultaneous dual polarization on receive, together with the necessity of containing or even reducing the cost, have brought to a different organization of the electronics of the SAS. This challenge can be faced through a novel design, based on technologies at the state of the art.
The CSG-SAR is equipped with an active phased array antenna, providing electronic beam steering capabilities in both the elevation plane and the azimuth plane. This antenna relies on 64 rows of 20 linear arrays each, arranged in three mechanical panels, in line with the CSK-SAR design (Figure 16). A modular approach is adopted in order to facilitate the production phase. This approach is founded on “macro-tiles”, which are complete active antennas equipped with 256 single channel TRMs (128 for each linear polarization) and including all the relevant electronics to drive and supply the modules and the radiating boards connected to the modules.
From a functional point of view, the basic element of the SAS is the EFE (Electronic Front End). Each EFE includes 32 TRMs operating at single polarization, assembled on two columns of 16 modules each. Furthermore, two lateral power cards implement the power conditioning for the two columns of TRMs, including storage capacitors and implementation of switching commands, while the central digital card, FPGA based, drives the modules in terms of amplitude and phase settings and selection of Tx and Rx polarizations. Finally, two radiating boards are directly mounted on each EFE to radiate the signal generated by the TRMs and to receive the field scattered by the targets on ground.
One macro-tile consists of 8 EFEs. The electronics which complete the macro-tile comprise 4 TPSUs (Tile Power Supply Units), 4 TTDLs (True Time Delay Lines) and 1 TCU (Tile Control Unit).
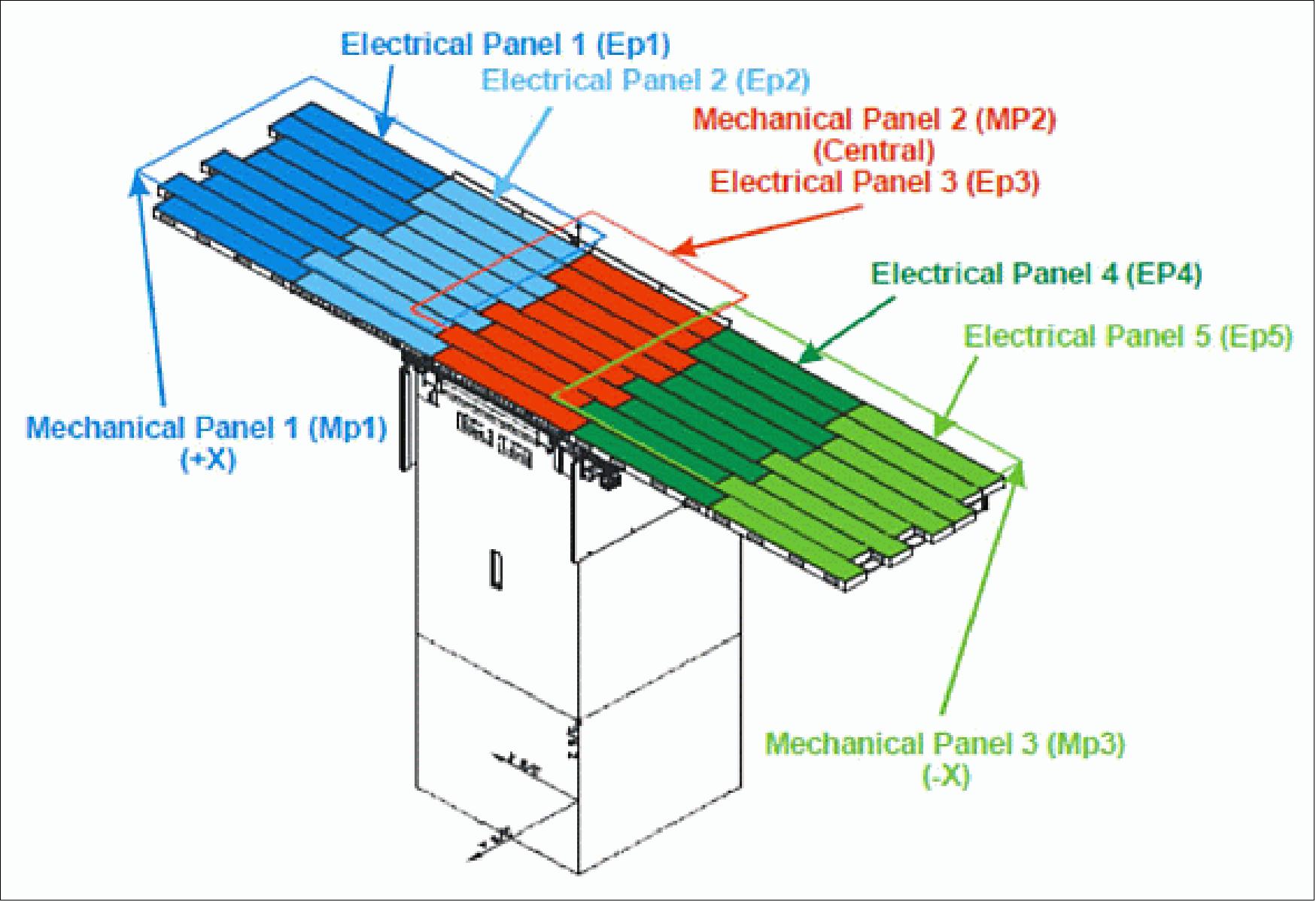
The architecture of each TRM (Figure 17) comprises a hybrid circuit containing a receiving chain, a transmitting chain and a common control section. The Tx and Rx chains are connected to the antenna port through a circulator.
The Control Block consists of a core-chip, which integrates on a single MMIC: a 6 bit step attenuator, a 6 bit phase shifter, Tx and Rx amplifiers, 6 RF switches and the RF interfaces towards the EFE Rx combiner and Tx divider. The switches are used to select the operative condition of the TRM, i.e. either Tx or Rx or calibration. The receiving chain of each TRM includes a LNA and a limiter made of a distributed circuit based on PIN diodes, while the transmitting chain comprises a driver amplifier and a HPA(High Power Amplifier) section, both built on a single MMIC. In order to support a complete calibration of the module, a coupler is inserted between the circulator and the antenna port. The signal coming from, and going to, the coupler is managed by the MMIC switches included in the core-chip. The temperature sensing block relies on a thermistor, which is read by the EFE digital section in order to compensate versus temperature all the TRMs of one macro-tile.
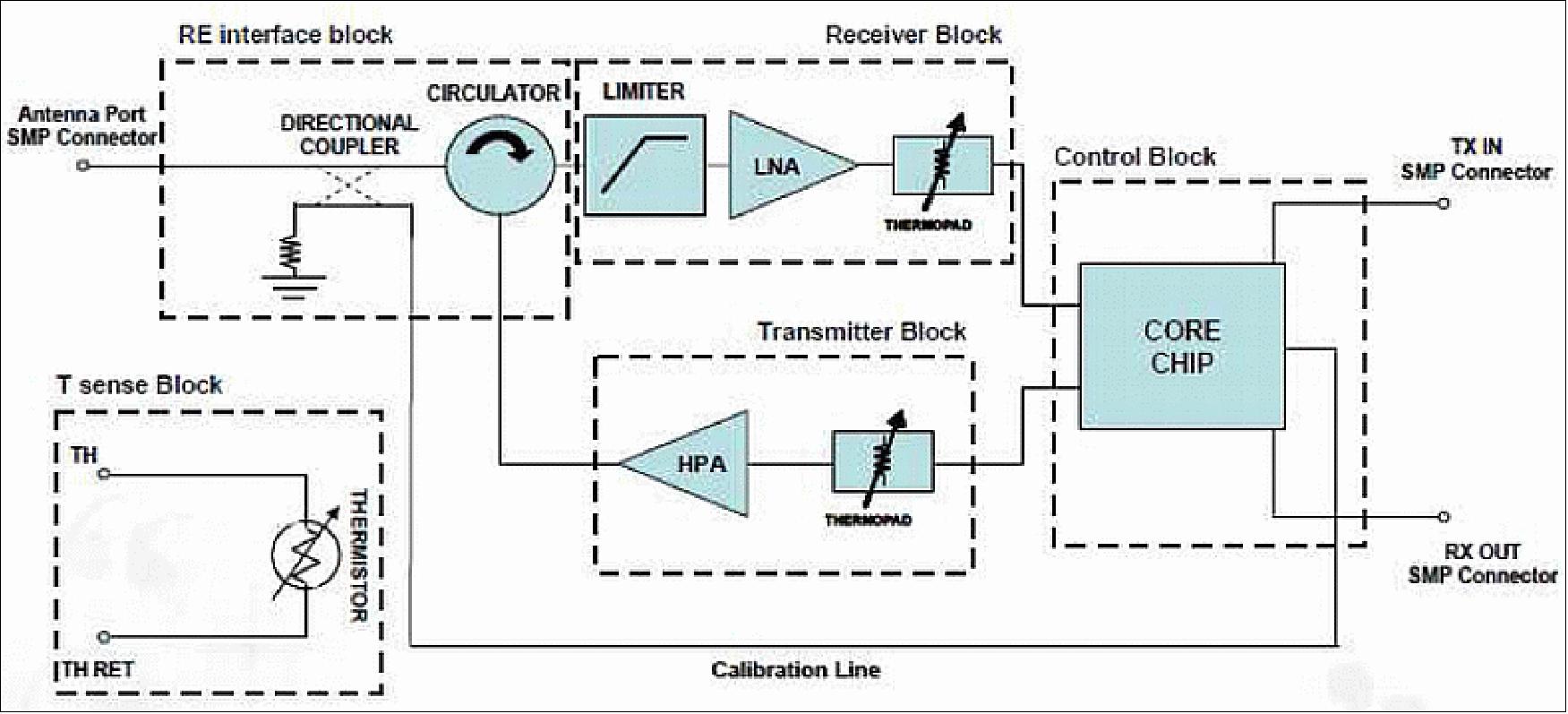
The radiating board is based on the same technology developed in the frame of CSK, which guarantees low mass, low cost, good performance, but a new design of the radiating patches and of the RF dividers have been carried out to enlarge the bandwidth and improve the cross polar performance in order to comply with the requirements for the dual polarization modes. Each radiating board includes 8 linear arrays working on double polarization, assembled into a single subassembly.
The TTDL consists of three RF hybrids and a digital power card to supply and drive the RF hybrids. The three hybrids, one Tx and two Rx, include 5 digital delays from 0.5 λ up to 15.5 λ. Moreover each RF path is provided with RF amplification and fine amplitude & phase tuning for temperature compensation.
The TCU is used to drive the settings of all the TRMs of the 8 EFEs (Electronic Front Ends) and of the 4 TDs. This unit is internally redundant in order to guarantee high reliability performance to the whole macro-tile.
Ground Segment
The CSG (COSMO-SkyMed Second Generation) GGS (Global Ground Segment) will be able to manage a constellation of 6 satellites (2 CSG satellites plus to 4 CSK satellites). From an operational point of view, the CSG GGS will grant a unique working environment for each operational role and operative profile. In particular, concerning the team organization structure, the basic assumption is to reuse the CSK system already deployed using the same management rules. This represents the reference baseline for all analysis and trade-offs that will be executed to identify the technical and/or organizational solutions allowing the optimization of the operational cost of the integrated system. 30)
A dedicated interim plan establishes the operational activities during the transition phase that starts during the development phase and stops with the completion of the in-flight commissioning. This plan harmonizes the activities required to deploy the CSG system and those already foreseen in the frame of the CSK MCO (Mantenimento in Condizioni Operative) contract in order to minimize the impact on the CSK operations and services.
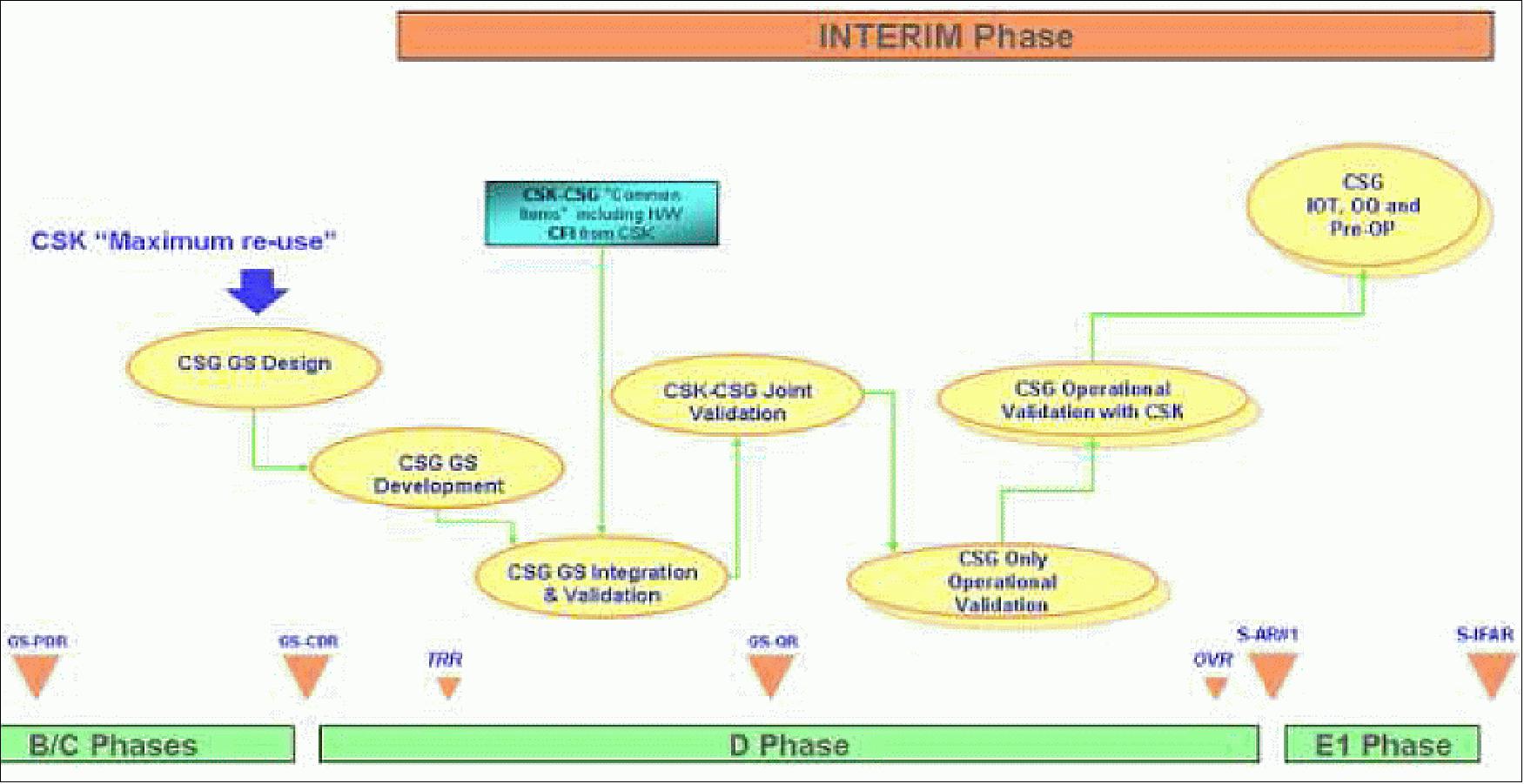
Design concepts:
Since the CSK system is already operational, the first activity carried out for the CSG design phase has been the analysis of the new CSG system requirements and their impacts on the existing architecture. The new functions and the upgrading of system functionalities/performances have been analyzed and introduced in the CSG architecture, considering the objective of reusing the existing asset as much as possible.
Therefore, although the system architecture definition process generally applies a top-down design approach, a parallel bottom-up approach has been considered to take into account the physical elements already developed and operating. This iterative process guarantees, from one side, the implementation of the functions/performances detailed in CSG system requirements and from the other side the minimization of the changes necessary to meet the above requirements.
Figure 19 illustrates the approach followed for the design of the CSG mission at both architectural and operational level, showing the integration with CSK.
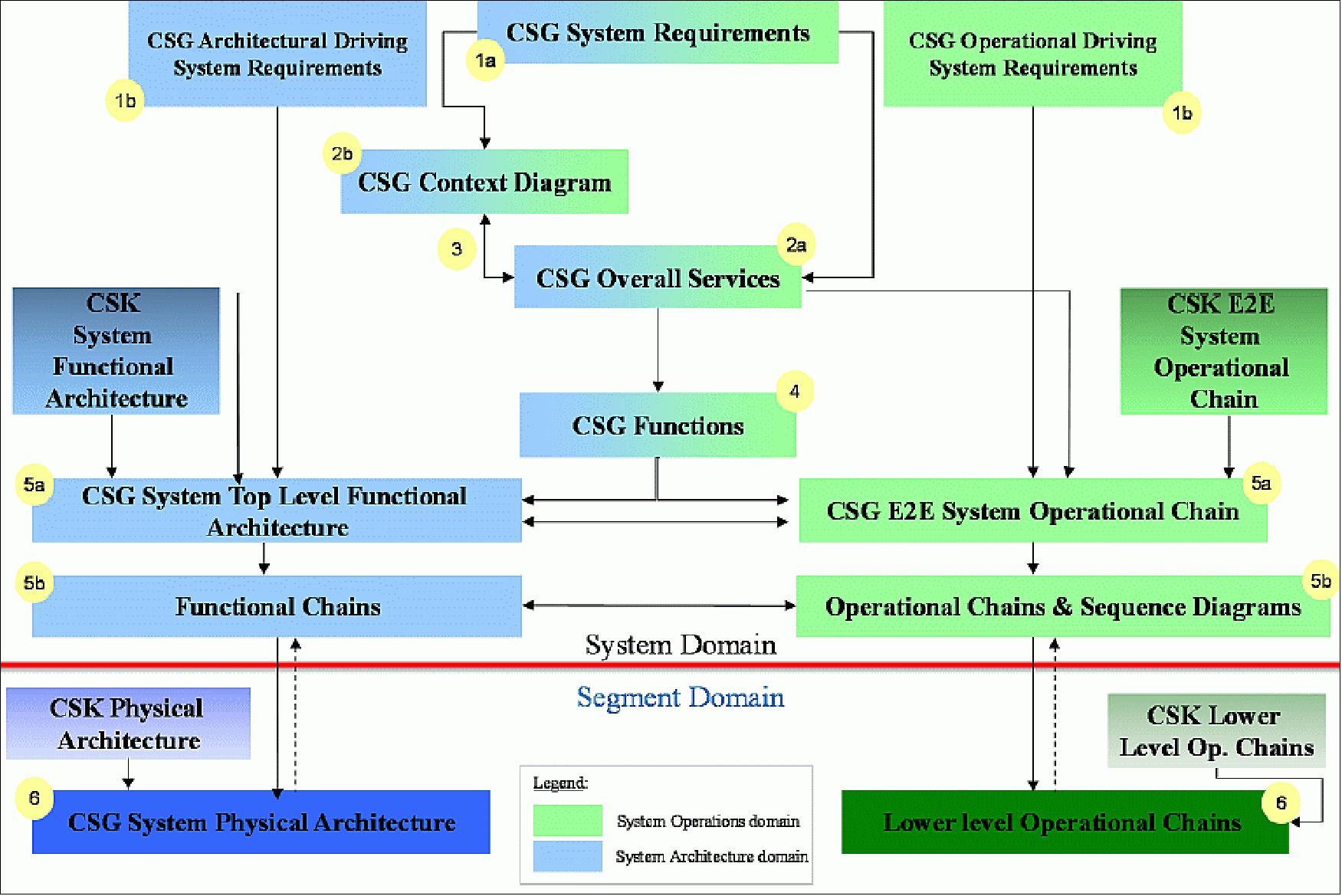
The key feature of the Architecture/Operations definition process is represented by the identification and definition (step 2a) of CSG’s Overall Services. These are defined as follows: The CSG overall service is a mean of delivering value to a consumer through a provider, by easing outcomes the consumer (e.g. the customer) expects to achieve. The overall service can be composed of a number of functions providing the capability to satisfy the stated consumer’s need or objective.
In the frame of COSMO Second Generation (CSG), an overall service is exchanged between providers and consumers identified at system level, according to the system context diagram (thus exchanged between the CSG system and its external entities). Lower level (e.g. segment level) value is exchanged by means of functions. The overall services’ analysis is influenced by both, the CSG’s system requirements, which address both certain specific services and – above all – the mission objectives, and the CSG’s system context diagram. The latter, defined on the basis of CSG’s system requirements too (step 1a, which is conducted in parallel with the service definition) and common to both operational and architectural domains, allows a mapping of one or more overall services towards a couple provider/consumer. To this aim, an iterative activity between identified services and context diagram (step 3 in Figure 19) serves as a compatibility check to guarantee that:
• for each overall service, both a provider and a consumer are identified
• vice versa, every provider/consumer couple exchanges an overall service.
The successive step (number 4 in Figure 19) consists in analyzing each overall service so as to derive the functions that allow its satisfaction. In this frame a function is defined as follows: CSG’s function, alone or as part of a set of functions, composes an overall service and provides so the capability to satisfy the consumer’s need or objective, thus the service provided is as expected.
The next step in the architectural design is then dedicated to the definition of the system functional architecture (step 5), defined as follows: System functional architecture: Decomposition of the system into functional chains and functions that, when all are fulfilled, completes the overall system mission, through a system services accomplishment. In addition, the functional architecture identifies the interfaces and the data exchanged among different functions.
CSG and CSK Common Ground Segment enhancement:
The CSG GS (CSG Ground Segment) is upgraded from the existing CSK GS to complement the Space Segment innovations necessary to manage, control, and utilize a System with such an increased performance. Moreover the CSG GS ensures the full architectural integration between the ‘old’ CSK and the new CSG ground architectures and capabilities, necessary for a smoother transition first, and an optimal conduction of both the systems during the nominal operative phase. 31)
The key concept is that both space components will actually operate as they were constituting a sole, fully integrated space segment. Furthermore, the ‘new’ CSG GS will sustain all the transition process that, while supporting CSG system deployment and qualification, will still maintain the CSK functionalities and operations in the both Defence and Civilian domains.
The Users interact with the Ground Segment by means of Multi-Mission Interface management, accessing different types of Services for requiring a new acquisition and standard product:
- CSK Service: the products already provided by the CSK mission
- CSG Service: the products provided by the CSG mission
- Specific Mission (Optical or SAR)
- Generalized Service: equivalent (CSK and CSG mission) or combined products (multi-mission).
The increased flexibility of CSG system is reflected also in an evolved Production Manager, improving the capacity of product direct delivery, the implementation of an unique multi-mission archive and catalogue facility (avoiding an archive duplication),the management of National Eye Only (NEO)/Non-NEO catalogs distributed among the Partners.
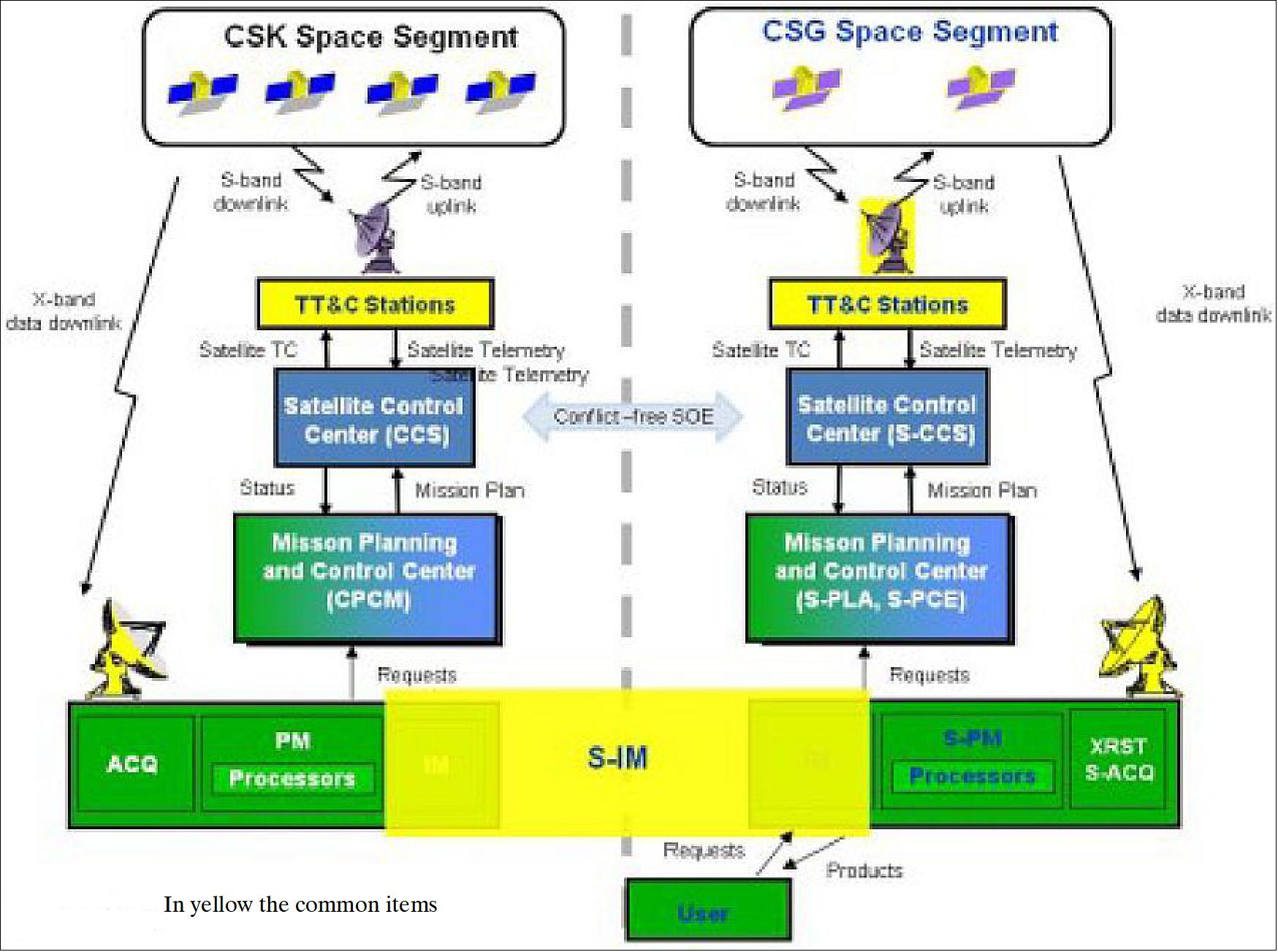
Calibration & Validation:
Being part of the end-to-end system design, the definition of CALVAL (Calibration and Validation) for the CSG integrated system, follows the same approach by taking into account drivers and bounds, most of all tied to the new challenging requirements. Keeping in mind the major constraints of continuity, maximum reuse and cost reductions, the new demanding needs of the CSG mission in terms of image quality performances led then to the evolution of CSK Calibration and Validation functions and capabilities (CSK CALVAL). 32)
The upgrade of CSK CALVAL toward a multi-mission CALVAL is guided by the lesson learned, the new SAR operative modes (e.g. Dual pol and Quad pol capabilities) and the new performances foreseen for CSG (e.g. geolocation accuracy).
According to the CSK design, based on a SECF (SAR Engineering Calibration Facility) and external calibrators, the new architecture foresees also the introduction of two new layers, the former technical and the latter operative, which embrace them.
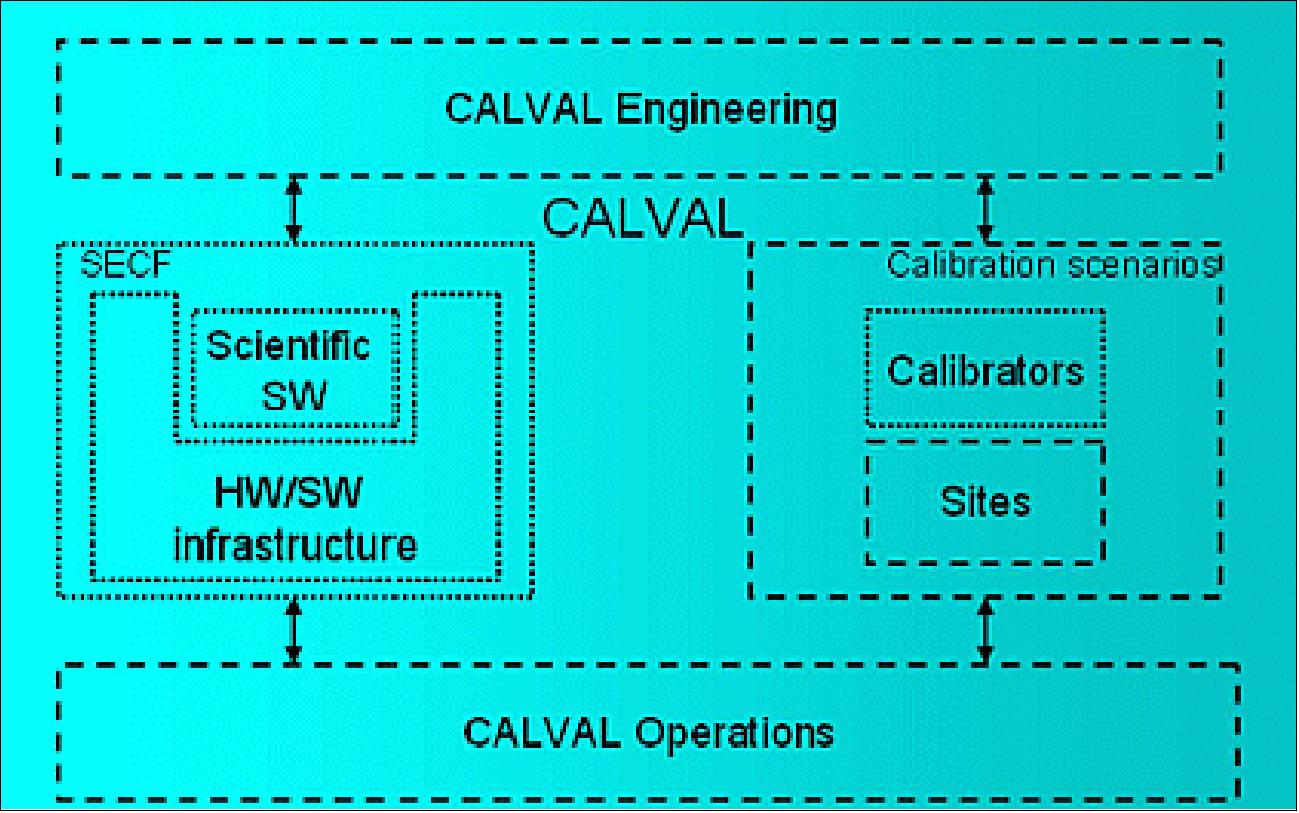
In particular the CALVAL engineering and operations have been defined. The former is in charge of doing all the not standard added-value activities with the aim to guide, coordinate and make decisions about all the calibration aspects. On the other hand all the manuals, procedures and operations concerning SECF (e.g. product management and processing) and calibrators (e.g. pointing) have been gathered in the CALVAL operations. For the sake of completeness, the distributed targets (e.g. Amazon forest) have also been included in the architecture. Both calibrators and sites are now gathered in the general concept of calibration scenarios. It must be remarked, that all these aspects were already embedded in CSK CALVAL, but the importance acquired during the operative phase led to clear those concepts up.
From a technical point of view, the existing elements of CSK CALVAL (SECF and calibrators) are now under analysis to design and implement all the modifications needed for the new multi-mission scenario. In particular, the evolution of the existing algorithms as well as the development of new processors is subject of the present activities. New aspects to take into account are the handling of new products with new characteristics and higher data volume (e.g. dual polarization). Moreover, from a calibration point of view, the required enhanced performances of geolocation led to the definition of dedicated models, as well as to the implementation of accurate analysis tools for the performance assessment and the generation of the related calibration tables. Concerning the calibration scenarios, new models of passive calibrators have been developed with the aim to improve radiometric and geometric accuracies. Moreover new attention has been paid on site characterization and selection.
Like with CSK, CSG CALVAL will be in charge of all the verification, validation and certification activities. The lessons learned acquired during the monitoring of the CSK constellation are contributing to a continuous upgrade of models and tools. All the enhancements foreseen for CSG mission are then the results of a progressive process of observation, understanding and improvement.
IEM (Interoperability, Expandability and Multisensor) Principles in COSMO-SkyMed
The IEM capabilities aim to support cooperation scenarios both among different CSK GS (Ground Segment) instances and between CSK and other EO systems. Interoperability is intended as the capability of two or more entities, at national or international level, to exchange information and data using a specific and agreed protocol (Ref. 12).
Expandability is the capability to cooperate with other EO systems by importing/integrating other EO system components or by exporting CSK components according to some defined interfaces (messages and dynamic behavior). The expandability can also be thought of as the system scalability aiming to ensure that:
• the CSK GS and the Integrated Logistic Support and Operations (ILS&OPS) Segment can be expanded to include: (i) up to six civilian UGS (User Ground Segment) instances; (ii) up to six defense UGS instances; (iii) up to six Distant Cells per UGS; (iv) a number of MAPS (Mobile Acquisition and Processing Stations) per each UGS deployed
• the UGS can be expanded in terms of processing capability in order to meet higher production volumes in case of increase of the production requirements
• the number of external stations (i.e. X-band acquisition station and S-band TT&C station) can be expanded within predefined limits upon Customer’s request and such an expansion can be managed without major modification.
The Multisensory feature (Multi Sensor / Multi Mission) allows to manage data originated by two separate missions or by different sensors of the same mission according to some constraints. With this objective, two major cooperation paradigms have been identified: Cooperation by Federation and Cooperation by Expansion.
5) Cooperation by Federation is granted through the exchanging of services according to agreed interfaces/protocols. The Federation does not require integration of physical components. This kind of integration provides the possibility of accessing the catalogue of Partner’s EO systems, searching for given products, ordering them, and receiving the queried products. Reciprocally, CSK allows the same type of access to Partner’s EO systems. This kind of interoperability is implemented basically through gateways which allow the access to Partner’s data through the CSK catalog Interoperability Protocol (IOP) developed according to CEOS guidelines.
6) Cooperation by Expansion between two EO systems is achieved by exchanging physical components, in a general sense hardware/software sub-system, to allow the EO System A to access to services/data made available by EO System B. It is worth noting that this cooperation expansion can be treated at two different levels:
• The expansion within the CSK boundaries by scaling up the system by UGS replication. In this case always the CSK interface shall be used.
• The expansion by providing CSK components/sub-systems to other EO systems or by integrating in CSK components/sub-systems provided by other EO systems.
In CSK both kinds of cooperation have been realized basically exploiting the Interoperability and Expandability capabilities. Partnerships and interoperability with foreign EO systems is also felt to be a primary characteristic of the COSMO-SkyMed System.
The COSMO-SkyMed System has been designed such to provide Interoperability, Expandability and Multi-Mission (IEM) capabilities with respect to:
• additional Defence and Civilian Partners
• other EO Systems/Missions – SAR- based
• other EO Systems/Missions – Optical-based.
These characteristics have triggered international cooperation programs, aimed at providing end-users with “added-value” products and services, and world-wide distribution of commercial COSMO-SkyMed data and services.
System IEM (Interoperability, Expandability and Multi Mission) requirements with partner EO Systems strongly affect the system operational concepts, operational scenarios and chronologies. Operations have been conceived to fulfill these requirements, applicable in different modalities to Defence and Civilian domains.
In fact, the dual use leads to a more complex system, able to manage at least two different classes of users (Defence and Civilian), with different priorities, privileges and more generally properties. In particular, it implies a complex scenario which guarantees on one side the security standards adequate for military use and, on the other side, provides the characteristics of flexible connectivity and information access necessary for civilian use. As such, the dual system interfaces fulfill the contrasting civilian and military utilization needs providing two classes of separate and differently protected interfaces respectively to:
- Unclassified Domain, protected at commercial standard level for civilian utilization
- Classified Domain, protected at military standard level. This domain includes Defence User Ground Segment, fully dedicated to military exploitation (national or international), and dual Ground Segment centers which treat both civilian and military information.
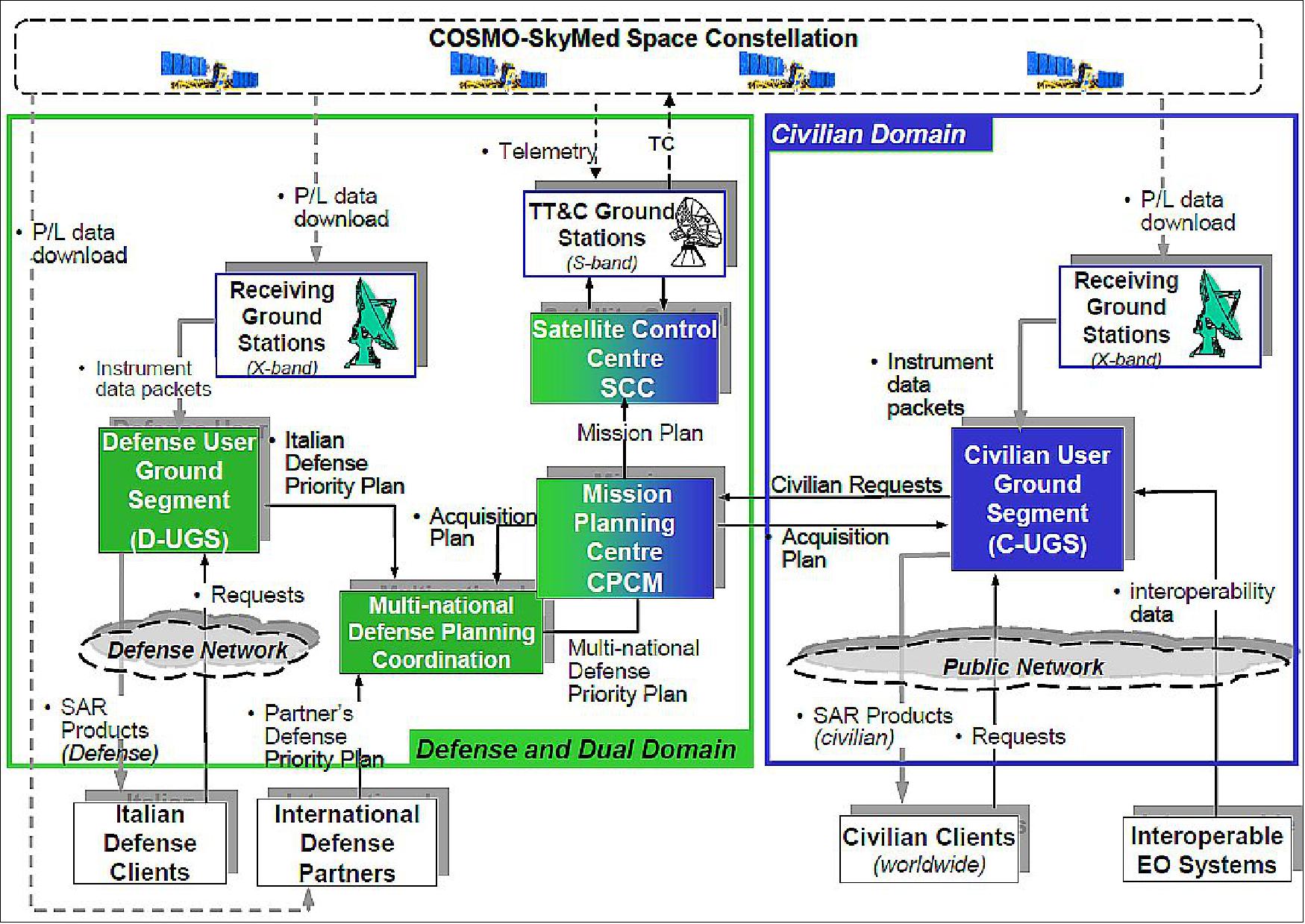
CSK (COSMO-SkyMed) Expansion & Federation:
CSK has been built-up by exploiting the IEM in a cooperation by expansion scenario. Every additional element is a properly customized and tuned replication of a part of the Italian Civilian or Defense UGS (User Ground Segment). This is the approach used to include in the Defense UGS:
1) a set of Italian Distant Cells
2) 1 Italian Defense-MAPS (Mobile Acquisition and Processing Station)
3) 1 French Defense UGS (User Ground Segment) 33)
The Civilian UGS enlists:
1) 1 Civilian-MAPS
2) Until a maximum of 20 1G TUP (Terminale Utente Portatile, Portable User Terminal first architecture/generation). 34)
In the Civilian context, a HMA (Heterogeneous Mission Accessibility) layer exposing an OGC (Open Geospatial Consortium) catalog interface to external clients was also added. It manages access to metadata records of the three supported providers (CSK, DigitalGlobe and GeoEYE). In particular, access to CSK metadata is performed through an Interoperability Protocol software adapter layer. In this case the expandability has been realized as cooperation by federation enabling partners access to CSK metadata.
CSG COSMO Plug-In Approach:
Both CSK and CSG GS are multi-mission and since the beginning it has been thought to support both different SAR (e.g. CSK and CSG, SIASGE – Italian-Argentinean Cooperation) and Optical (e.g. OPTSat 3000, a military mission) missions. For this reason, the CSK IOP (Interoperability Protocol) will be enhanced to become multi-mission including both CSG new parameters and optical specific parameters. To allow this capacity both the catalog and the ordering protocol (CSG ICD) are based on OGC standards.
Moreover, in the CSG UGS, in order to support expandability at finer-grained level and not based on the full or almost full UGS replication largely exploited in the CSK scenario, it has been thought a plug-in based approach. The plug-in approach defines a framework that will allow CSG UGS to exploit the add-in functionalities. The Plug-in concept introduced is based on the OOP (Object-Oriented Programming) interface concept (widely used, for example, in the Java programming language).
The OOP interfaces define a standardized set of commands that a class will obey. The commands are a set of methods that a class implements. The interface definition states the names of the methods and their return types and argument signatures. Therefore, in OOP interfaces there is no executable body for any method. The implementation of the method’s body is in charge of each class that implements the interface. In its most common form, the interface defines which logic functionalities and activities a class has to perform, but not how. The classes implementing the interfaces describe how to perform the functionalities. These classes are the plug-ins that shall be implemented.
According to this approach the system internal servers, in particular the CSG Interface Manager (S-IM) servers which are devoted to fully manage information flow through all interface of CSG UGS, shall access the needed service (catalog, ordering or feasibility/programming), using always the same Common Methods ignoring the protocol of the server to be addressed. The link between the Common Methods and the protocol supported by the server is in charge of the plug-ins (i.e. the defined classes). Obviously S-IM is in charge of instantiating the proper plug-in depending on the service and on the mission involved. S-IM shall be provided with a plug-in dispatcher, in charge of instantiating the correct plug-in for the mission and the needed functionalities.
According to the mission and the functionality, the correct plug-in shall be instantiated. The plug-in approach allows the S-IM to offer three types of interfaces:
• GS CSG ICD interfaces implemented by means of S-IM plug-ins
• GS CSK ICD interfaces implemented by means of S-IM plug-ins
• CJI (Common Java Interfaces), offering a set of API allowing expansion to Foreign Mission (FM) GS protocols through dedicated plug-in to be developed.
CSG Cooperation by Federation:
This section describes how the current CSK GS and in perspective CSG GS expandability capabilities support interoperability with different EO Ground Segments (generically referred as FM GS (Foreign Mission Ground Segment).
The FM is expected to support, through its defined interfaces, the following functionalities:
• Feasibility Analysis: to check the possibility to perform sensing on the selected Area of Interest during the selected Time Window and according to the chosen mission/satellite acquisition parameters
• Check-Conflict (National Harmonization): to verify if any sensing request is conflicting with any others and submit the final harmonized Service Request list to the FM GS
• (International) Harmonization: to verify and harmonize the Service Request lists coming from the different Partners
• Acquisition Planning follow-up: to be used by the FM to provide the S-IM with the planning of its acquisition requests
• Ordering: to allow S-IM to submit production requests towards the FM when receiving confirmation that the acquisition is planned
• Ordering follow-up: to allow the FM to keep S-IM informed about the progression of the order
• Delivery: the CSG GS is the only responsible for the delivery to the final user. For this reason an interface shall be defined on FM so that it can provide the CSG Production Manager (S-PM) with the product to be delivered.
Obviously, different scenarios could be put in place according to the capabilities offered by the Foreign Mission (e.g. International Harmonization might not be supported at all).
Regardless of the capabilities supported, there are three possible expandability scenarios according to the alignment degree between the interfaces supported by CSG and the Foreign Mission ones.
• The first scenario fully exploits the CSG scalability and expandability that allows supporting the FM, with neither architectural modification, nor further development, nor new plug-in deployment, by providing interfaces developed in compliance with the CSG paradigm (Programming Request, Acquisition Request and Data Take Opportunities) and supporting the CSG interfaces.
• The second scenario exploits the CSG scalability and expandability that allows supporting the FM, with the development and the deployment of the plug-ins, providing interfaces developed in compliance with the CSG paradigm (Programming Request, Acquisition Request and DTO) and supporting only their specific interfaces (FM ICD).
Obviously, intermediate scenarios can be derived from the previous two if the FM owns some interfaces which are compliant with CSG ones and some which are not, and/or some functionalities are provided and others are not.
• The third scenario has a unique access point for any interaction exchanged between the two missions. In this scenario, there is not any new plug-in to be deployed in the S-IM server.
CSG UGS Expansion Scenarios:
As explained in the previous section, the CSG system is expandable even through the replication of the UGS. In this regards, it is also possible to tailor the UGS services according to its main features:
• Request definition and feasibility analysis
• Request ranking and harmonization
• SAR data acquisition through local antenna
• SAR data processing
• Product catalogue updating, maintenance and browsing.
There are five different possibilities to access CSG system through the definition of “Partner XUGS” modulated according to the partners’ characteristics and needs. X in “X-UGS” stands for either Defense or Civilian.
1) The first type of X-UGS expandability is characterized by a full autonomy of the Partner to which all the listed UGS services are provided.
2) The second type of X-UGS expandability is characterized by the lack of capability to acquire directly SAR data (i.e. the local antenna is not available); in this case the SAR data are received from external antennas.
3) The third type of X-UGS expandability is characterized by the absence of acquisition and processing functionalities; in this case these services can be provided by Italian X-UGS through a Partners shared-key policy.
4) The fourth type of X-UGS expandability requires the Partner X-UGS to manage the Service Request up to the Feasibility Analysis, whereas the Italian X-UGS is in charge of managing the ranking, addressing acquisition and processing on behalf of the partner. The partner maintains the full control of the catalog. Also in this case, a shared key management mechanism between the two Partners is needed.
5) Similarly to the fourth case, also the fifth type of X-UGS requires the Partner X-UGS to manage the Service Request up to its submission.
The Italian X-UGS is in charge of managing both procurement and ranking, by addressing acquisition and processing on behalf of the Partner. The Partner retains all the catalog features. Also, a key-sharing mechanism is required.
Actual Cases
F-DUGS (French-Defence User Ground Segment)
F-DUGS is an example of expansion “by-partner” of the COSMO-SkyMed System, which means an additional complete partner User Segment, developed for providing French defense users with access capability towards COSMO-SkyMed, in the frame of the ORFEO cooperation program.
The F-DUGS center is located in France and became operative in 2010. From an architectural point of view, F-DUGS is an additional instance within COSMO-SkyMed Defence user centers, equipped with all necessary technical means and solutions for guaranteeing security, integrity and confidentiality constraints, and provides end users with capabilities for:
• Request programming and pre-feasibility analysis, including request harmonization with other defense partners
• Satellite data acquisition, by local antenna or by means of COSMO-SkyMed External Stations
• Data processing and distribution
• Archiving and cataloguing of all generated products and related auxiliary data.
The F-DUGS center is autonomously operated by French personnel, and it is operatively integrated with COSMO-SkyMed System by means of a well-defined set of interfaces and processes, which allows the center to be informed on system chronologies changes, on relevant on-board activities. The center is also inserted in the system maintenance loop, and upgraded any time a relevant new SW patch is released. — Such result comes up directly form the “built in” expandability capabilities natively designed in COSMO-SkyMed.
MUSIS (MUltinational Space-based Imaging System) for surveillance, reconnaissance and observation
MUSIS is an endeavor launched by several European nations to bring together the coming generation of military and dual-use space-based EO (Earth Observation) systems. The main objective of MUSIS is to provide continuity of service of the current generation of optical and radar satellites and to ensure adequate access of the partners to space systems which will enter into service in the coming years.
In this frame, France and Italy have defined a ground federating system called CIL (Common Interoperability Layer), which aims at interoperating the French ”Composante Spatiale Optique” and the Italian CSG systems. The CIL will provide Italy and France with an easy and reliable access from their national own space system to optical and radar images which will be acquired by two Earth observation systems.
The CIL design foresees the development of an advanced bridge to be placed in between the user ground segments of the two space systems, which are to be designed with enhanced interoperable, flexible and modular interfaces. The CIL will enable operators from one nation to order image products from the other nation’s system, task the satellites of the other nation, receive the image products (both new acquisitions and stored in common archives) and store them in a fully secure way. The personnel needed to operate the CIL will be kept at minimum, thereby minimizing lifecycle costs. The CIL will be based on a suite of services (i.e. hardware and software either developed in common or made available by one nation to the other), standard format interfaces and a secured network.
The MUSIS CIL enables multi-sensor cooperation, with the following minimum objectives:
• allowing the access of Partners to both optical and SAR satellite capabilities
• reducing life cycle costs
• preserving confidentiality for requests and products.
SIASGE (Sistema Italo Argentino de Satélites para la Gestión de Emergencias - Italian/Argentinian satellite system for emergency management)
ASI and CONAE [Comisión Nacional de Actividades Espaciales, Buenos Aires, Argentina (National Commission on Space Activities)]have decided to envisage the SIASGE System, as integration of the COSMO-SkyMed and SAOCOM projects (complying with respective Programs/Project constraints), conceiving then the SIASGE as a double-band (X+L) SAR Mission, in order to provide the most adequate information for emergency management, environmental monitoring and resources management of land and see, among other important applications.
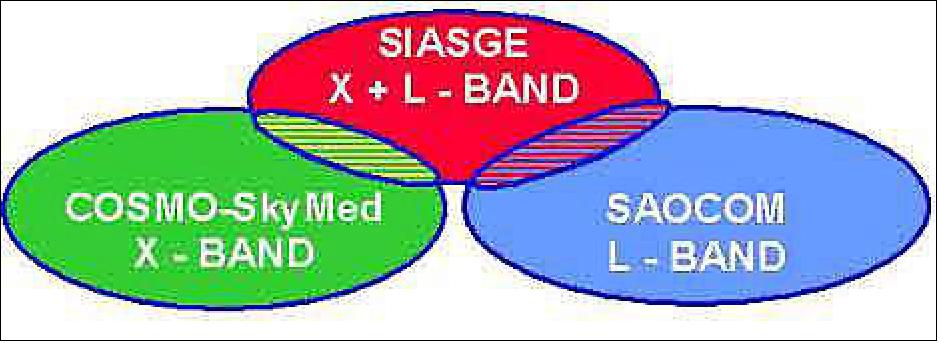
The main functional elements which are exploited by SIASGE System are:
1) The X-Band Component (COSMO-SKYMED-based)
2) The L-Band Component (SAOCOM)
3) A Common Ground Infrastructure with the aim of:
• interfacing SIASGE Users with capabilities outside the already available one (TBD)
• interfacing COSMO-SkyMed and SAOCOM, for standard product requests and reception with capabilities outside the already available one
• generating Joint Value added products
• exploiting and distributing Value added products.
According to the current MOU, the system deployment will take into account the existing proprietary Ground Segment facilities such as the ASI facilities in Matera and Fucino and the CONAE facilities in Cordoba. The SIASGE Common Ground Infrastructures shall be deployed in Argentina (Cordoba) and in Italy (Matera).
The generic schema for the definition of the architecture of the SIASGE common ground segment, is shown in the Figures 24 and 25.
The IEM (Interoperability, Expandability and Multi-Mission/Multi-Sensor) CSK key principles have been applied in several actual cases, such as for example the F-DUGS (French Defence User Ground Segment) and the Portable User Terminals. CSG, In June 2016 at the end of the design phase, has improved these characteristics to support several kinds of cooperation scenarios between the whole first + second generation system and other EO systems, making COSMO an “open” system in a broad sense.
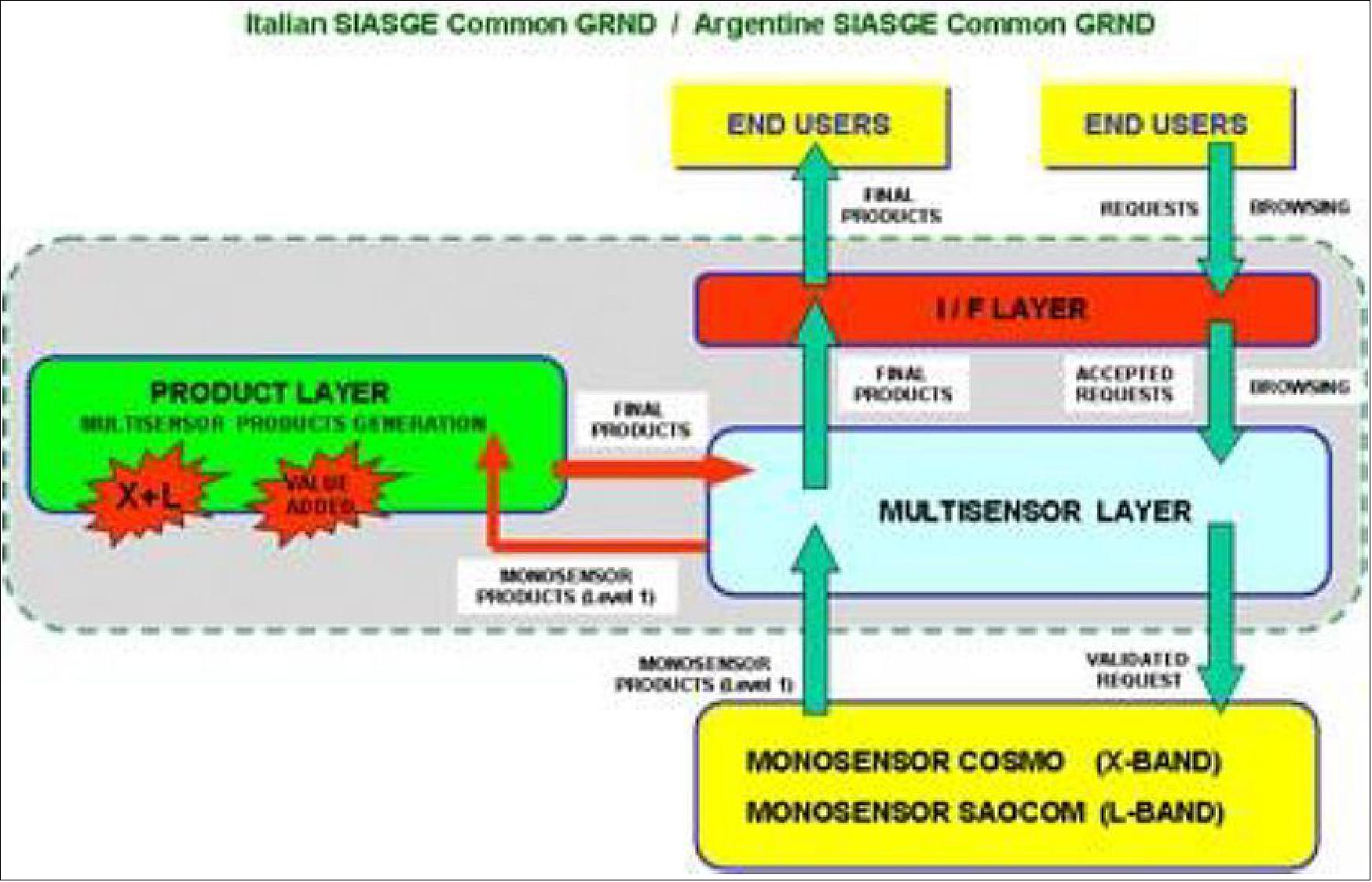
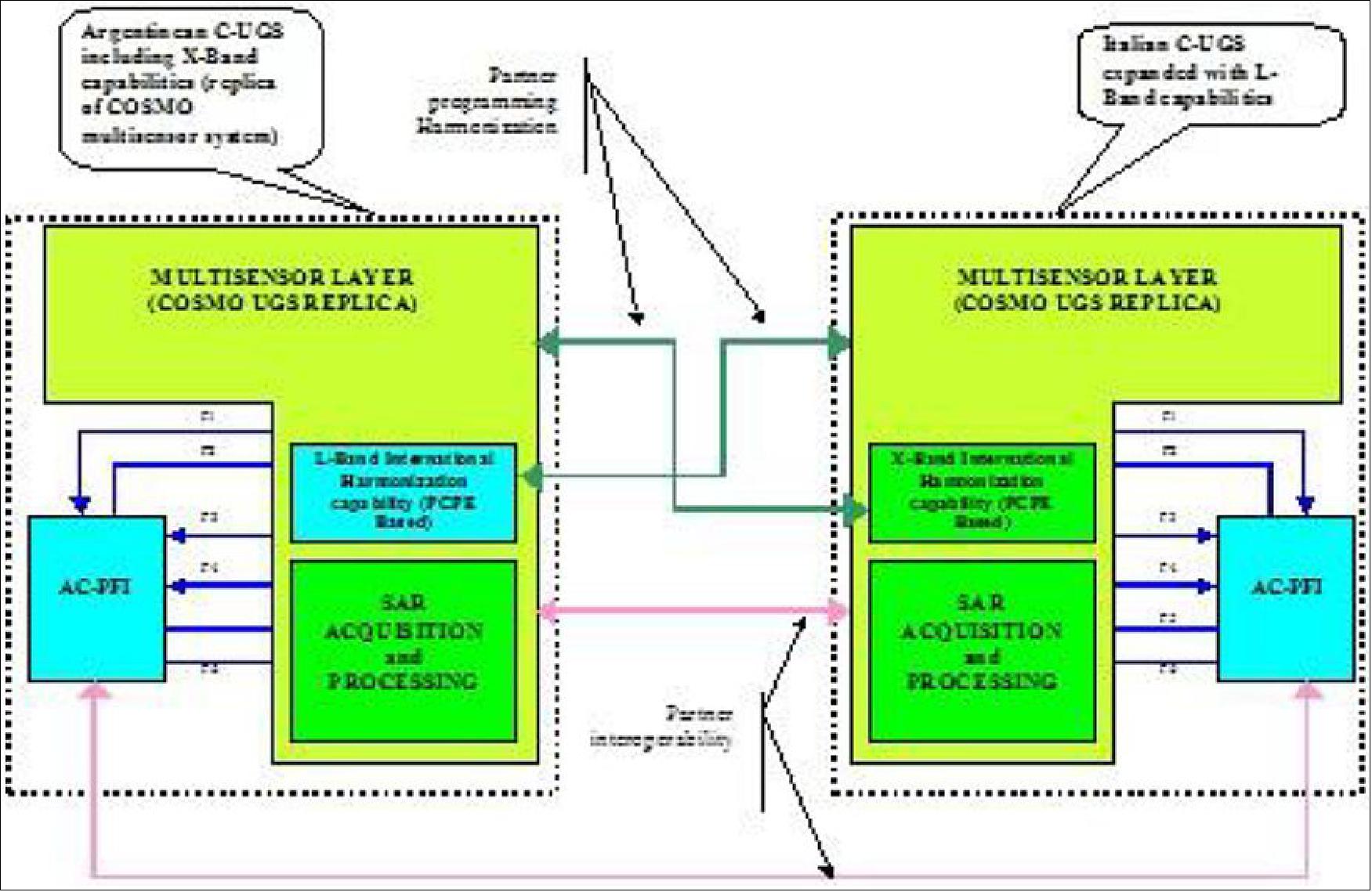
Figure 25: Preliminary architecture of the UGS (X+L band), image credit: ASI, CONAE
The COSMO-SkyMed Ground Segment is geographically distributed over a wide territory, including Fucino, Matera, Pratica di Mare and some external stations. 35)
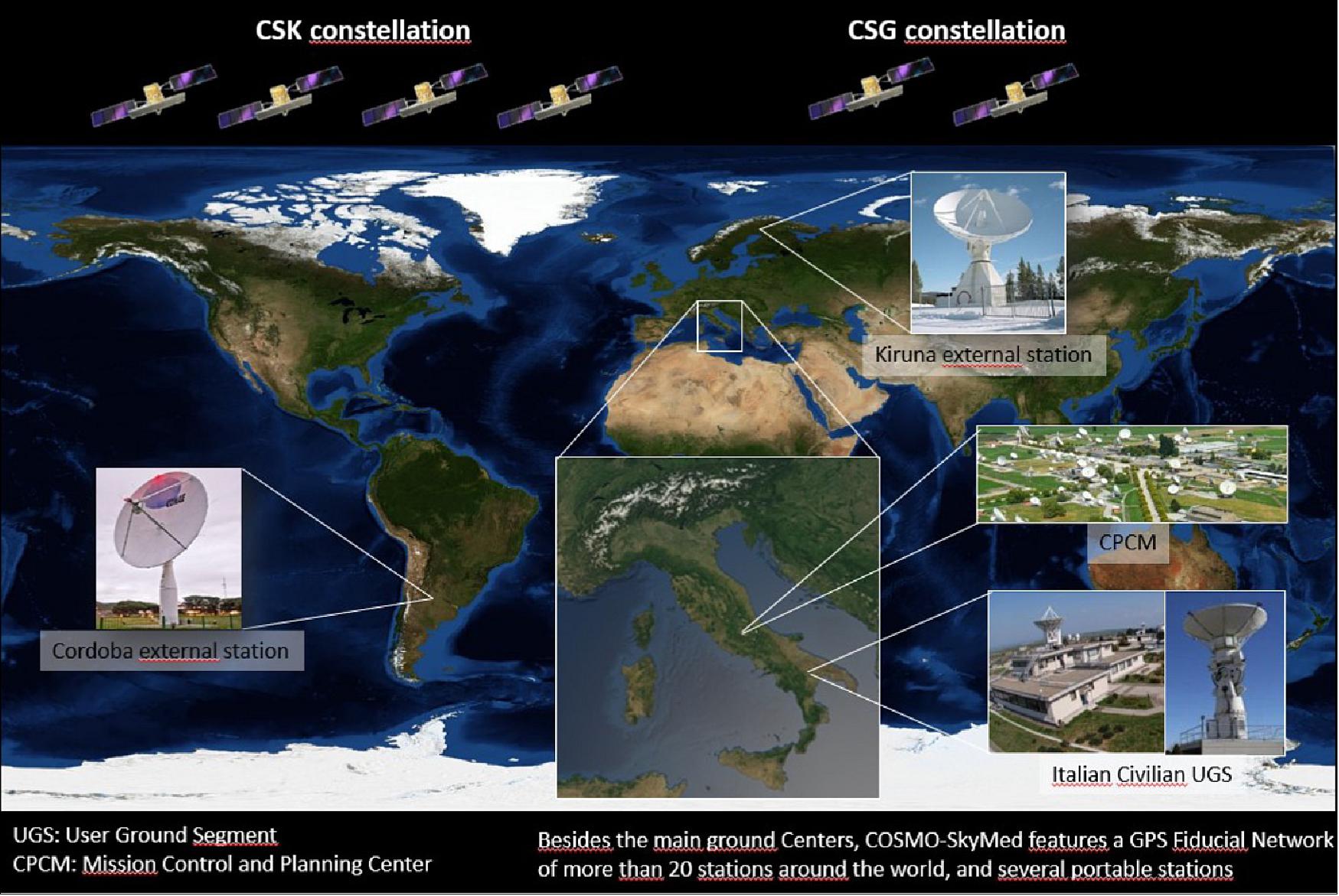
In addition, the GS uses a world-wide network of GPS receivers to improve performance requirements on the product Geolocation accuracy and the CSK GS includes mobile terminals (C-MAPS, TUPs and D-MAPS) for civilian and defense utilization.
The COSMO SkyMed top-level decomposition is made by the following Elements:
• the Mission Planning Center (CPCM), which is located in Fucino plain in Italy, is in charge of coordinating the on-board and ground activities, performing overall mission planning, allocating resources and solving conflicts;
• the Core Ground Segment (CGS), encompassing all those facilities devoted to manage the satellite constellation;
• the User Ground Segment, further composed by a Civilian and a Military center (C-UGS and D-UGS) which manages the user requests to acquire, archive, process and deliver the products data. Only within the CSK UGS, some Mobile Stations extend the UGS usage;
• the G/S Global network to provide communication services;
• a set of complementary elements grouped as Service Support Element (SSE) in CSK, and that in CSG have been pointed explicitly and managed individually (e.g.: External Station, GFN).
The improvements introduced by the CSG Ground Segment (that is a multi-mission system thanks to its enhanced features and therefore able to interact with the CSK Ground Segment) will allow to consider and interact with CSG and CSK as a single unique system, having the following main characteristics:
• an integrated operative environment,
• a set of products defined as equivalent and the capability to handle them in order to fulfil specified generalized services,
• an integrated management of the available resources.
The improvement of the Ground Segment with respect to the CSK system consists in the ability to handle and treat a massive amount of data in order to obtain more performing products in shorter response time and into a specific timing. This is somehow correlated with the improvement of the Space Segment , that in turn responds to the demand of new products and services defined by Defense, Civilian and Commercial Users.
At the same level of importance of the innovations, there are the constraints related to the ‘relation’ with CSK, all together referred as “CSK continuity”. It can be summarize in the following requirements:
• The CSK capability shall not be modified, for aspects related to the service implementation, qualification and certification;
• The compatibility vs CSK data shall be guaranteed: data and products generated by CSK and currently archived at UGS have to be accessible and interpretable, at format level by CSG;
• To provide an integrated COSMO-SkyMed (CSK & CSG) service: even if the two systems and the related performances are independent, the user request shall be processed in an integrated way, addressing it to the mission defined explicitly by the user/operator or to both systems.
Main drivers for this architectural solution are:
• keeping the operative continuity not critical: indeed both the planner and the control centre functionalities manage separately the 2 constellations
• keeping the AIV and qualification regression tests limited to the S/S interfacing the common layers
• minimizing the needs of re-certification, as the modifications are focalized on interfaces to the layer.
References
1) Mario Cosmo, Fabrizio Battazza, Francesco Caltagirone, Fabio Covello, Giuseppe Francesco De Luca, Roberto Formaro, Francesco Longo, Giancarlo Natale Varacalli, “The future EO ASI missions are based on SAR and hyperspectral sensors,” Proceedings of the 63rd IAC (International Astronautical Congress), Naples, Italy, Oct. 1-5, 2012, paper: IAC-12-B1.2.8
2) Gianni Casonato, Francesco Caltagirone, Giuseppe Francesco De Luca, Fabio Covello, Claudia Anita Maria Fiorentino, Manfredi Porfilio, Davide Di Domizio, Matteo Piemontese, Elvira Caliò, Anna Croce, Giuseppe Olivieri, Andrea Gallon, Diego Calabrese, Emanuele Giacomoni, Alberto Cenci, Alessandro Di Bona, “COSMO-SkyMed Seconda Generazione Development Status and Prospects relevant to Interoperability, Expandability and Multi Mission/Multi Sensor Capabilities,” Proceedings of the 63rd IAC (International Astronautical Congress), Naples, Italy, Oct. 1-5, 2012, paper: IAC-12-B1.6.8
3) Diego Calabrese, Anna Croce, Gerardo Spera, Roberto Venturini, Giuseppe Francesco De Luca, Stefano Serva, “COSMO SG, System Overview and Performance,” Proceedings of EUSAR 2014 (10th European Conference on Synthetic Aperture Radar), Berlin, Germany, June 3-5, 2014
4) Edmundo Scorzafava, Fabio Monaci, Enrico Zampolini Faustini, Mechelangelo L'Abbate, Pasquale Capece, Franceso Lumaca, Giovanni Campolo, Aniceto Panetti, Gerardo Spera, Patrizio Pavia, Silvia Occhigrossi, Simone Lunardini, Marco Anania, Roberto Venturini, Francesco Spadoni, Rita Roscigno, Flaviano Bagaglini, “COSMO SG, Spacecraft design and technological challenge,” Proceedings of EUSAR 2014 (10th European Conference on Synthetic Aperture Radar), Berlin, Germany, June 3-5, 2014
5) S. Mari, G. Valentini, T. Scopa, S. Serva, M. Porfilio, M. Cardone, C.A.M. Fiorentino, ”The MultiMission COSMO-SkyMed di Seconda Generazione Ground Segment,” Proceedings of the 14th International Conference on Space Operations (SpaceOps 2016), Daejeon, Korea, May 16-20, 2016, paper: AIAA 2016-2606, URL: http://arc.aiaa.org/doi/pdf/10.2514/6.2016-2606
6) Valerio Grimani, Alessandro Cricenti, Andrea Perrera, Mario Cossu, Francesco Caltagirone, Claudia Anita Maria Fiorentino, Silvia Mari, Manfredi Porfilio, Giuseppe Francesco De Luca, Stefano Serva, Paolo Inversi, Pier Giorgio Esposito, ”COSMO-SkyMed: From the first towards the second generation,” Proceedings of the 67th IAC (International Astronautical Congress), Guadalajara, Mexico, Sept. 26-30, 2016, paper: IAC-16,B1,2,9
7) Diego Calabrese, Flavia Carnevale, Anna Croce, Ignazio Rana, Gerardo Spera, Roberto Venturini, Chiara Germani, Francesco Spadoni, Flaviano Bagaglini, Rita Roscigno, Luigi Corsano, Stefano Serva, Manfredi Porfilio, Giuseppe Francesco De Luca, “New concepts and innovative solutions of the COSMO-SkyMed 'Seconda Generazione' System,” Proceedings of the IGARSS (International Geoscience and Remote Sensing Symposium) 2015, Milan, Italy, July 26-31, 2015
8) Patricio Pavia, Gerardo Spera, Roberto Venturini, Flavio Bagaglini, Simone Lunardini, Edmodo Scorzafava, Efer Miotti, ”CSG satellite design and performance,” Proceedings of EUSAR 2016, 11th European Conference on Synthetic Aperture Radar, Hamburg, Germany, June 6-9, 2016
9) Gianni Casonato, Michelangelo L'Abbate, Paolo Venditti, Flaviano Bagaglini, Luigi Corsano, Adriano Lupi, Rita Roscigno, Roberto Sigismondi, Davide Di Domizio, “High performances Data Handling And Transmission systems: the COSMO-SkyMed Seconda Generazione solution,” Proceedings of the 63rd IAC (International Astronautical Congress), Naples, Italy, Oct. 1-5, 2012, paper: IAC-12-B1.4.1
10) Diego Calabrese, Chiara Germani, Andrea Torre, Davide Rizzato, Stefano Frederici, Roberto Formaro, Giancarlo Natale, Claudia Facchinetti, ”CSG Status and New Solutions to Increase the Product Portfolio,” EUSAR 2018 (12th European Conference on Synthetic Aperture Radar), Aachen, Germany, June 4-7, 2018
11) ”Double Dealing by Arianespace: COSMO CSG x2 Satellites and Vega Launchers Ordered Up,” Satnews Daily, 28 Sept. 2017, URL: http://www.satnews.com/story.php?number=1955689138
12) G. F. De Luca , G. Valentini, L. Fasano, C. A. M. Fiorentino, M. Porfilio, T. Scopa, ”The Capability of COSMO-SkyMed Di Seconda Generazione to Support Cooperation Scenarios with Other Earth Observation Systems,” Proceedings of the 14th International Conference on Space Operations (SpaceOps 2016), Daejeon, Korea, May 16-20, 2016, paper: AIAA 2016-2421, URL: http://arc.aiaa.org
/doi/pdf/10.2514/6.2016-2421
13) ”The first COSMO-SkyMed Second Generation satellite has been launched successfully,”Telespazio, 18 December 2019, URL: https://www.telespazio.com/en/news-and-stories-detail/-/detail/
171219-the-first-second-generation-cosmo-skymed-satellite-has-been-launched-successfully?f=%2Fnews-and-stories-detail
14) ”Arianespace’s last mission of the year a complete success: COSMO-SkyMed Second Generation, CHEOPS, OPS-SAT, EyeSat and ANGELS now in orbit,” Arianespace, 18 December 2019, URL: https://www.arianespace.com/press-release/
arianespaces-last-mission-of-the-year-a-complete-success-cosmo-skymed-second-generation-cheops-ops-sat-eyesat-and-angels-now-in-orbit/
15) ”Liftoff for Cheops, ESA’s exoplanet mission,” ESA Science & Exploration, 18 December 2019, URL: http://www.esa.int/Science_Exploration/Space_Science
/Cheops/Liftoff_for_Cheops_ESA_s_exoplanet_mission
16) Stephen Clark, ”Soyuz • CSG 1 & CHEOPS,” Spaceflight Now, 7 October 2019, URL: https://spaceflightnow.com/launch-schedule/
17) Stephen Clark, ”Launch Schedule,” Spaceflight Now, 15 August 2018, URL: https://spaceflightnow.com/launch-schedule/
18) Enrico Zampolini Faustini, Matteo Piemontese, ”COSMO Second Generation — State of art technologies and system innovations for Earth Observation servicing excellence,” ASi Workshop, Rome, Italy, 13-15 November 2017, URL: https://bandiasi.almaviva.it/sites/default/files/attach/evento/02_asi_workshop_15-11-2017_csg_state_of_art_technologies_final.pdf
19) ”Exoplanet mission launch slot announced,” ESA, 23 November 2018, URL: http://sci.esa.int/cheops/60949-exoplanet-mission-launch-slot-announced/
20) ”Arianespace to launch a French astronomy mission in 2019: the EyeSat Triple Cubesat,” Arianespace, 9 January 2019, URL: http://www.arianespace.com/press-release/
arianespace-to-launch-a-french-astronomy-mission-in-2019-the-eyesat-triple-cubesat/
21) Jeff Foust, ”Falcon 9 launches Italian radar satellite,” SpaceNews, 31 January 2022, URL: https://spacenews.com/falcon-9-launches-italian-radar-satellite/
22) ”COSMO-SkyMed Second Generation data now open to users,” ESA News, 27 April 2021, URL: https://earth.esa.int/eogateway/news/cosmo-skymed-second-generation-data-now-open-to-users
23) Roberto Venturini, Francesco Spadoni, Renato Croci, Andrea Torre, Pasquale Capece, Francesco Caltagirone, Fabio Monaci, “COSMO SG, SAR Instrument Design,” Proceedings of EUSAR 2014 (10th European Conference on Synthetic Aperture Radar), Berlin, Germany, June 3-5, 2014
24) Roberto Venturini, Manuela Di Salvo, Chiara Germani, Andrea Olanda, Claudio Scarchilli, Matteo Soccorsi, Francesco Spadoni, Aldo Torrini, Pasquale Capece, Francesco Barletta, Antonio Delfino, “COSMO SG SAR,” Proceedings of the Advanced RF Sensors and Remote Sensing Instruments &Ka-band Earth Observation Radar Missions, (ARSI'14 & KEO'14), ESA/ESTEC, Noordwijk, The Netherlands, Nov. 4-7, 2014
25) Stefano Serva, Claudia Fiorentino, Fabio Covello, “The COSMO-SkyMed Seconda Generazione key improvements to respond to the user community needs,” Proceedings of the IGARSS (International Geoscience and Remote Sensing Symposium) 2015, Milan, Italy, July 26-31, 2015
26) C.A.M Fiorentino, S. Serva, S. Mari, M. Porfilio, ”COSMO-SkyMed Di Seconda Generazione Mission Requirement Refinement Process During Phase C,” Proceedings of the 14th International Conference on Space Operations (SpaceOps 2016), Daejeon, Korea, May 16-20, 2016, paper:AIAA 2016-2356, URL: http://arc.aiaa.org/doi/pdf/10.2514/6.2016-2356
27) Diego Calabrese, Flavia Carnevale, Vanessa Mastroddi, Manuela Marabucci, Giuseppe Francesco, De Luca, Claudia Anita Maria Fiorentino, Stefano Serva, Efer Miotti, ”CSG System Performance and Mission,” Proceedings of EUSAR 2016, 11th European Conference on Synthetic Aperture Radar, Hamburg, Germany, June 6-9, 2016
28) Roberto Venturini, Francesco Spadoni, Chiara Germani, Matteo Soccorsi, Claudio Scarchilli, Aldo Torrini, Pasquale Capece, Antonio Delfino, Francesco Barletta, Francesco Caltagirone, Marco Nardini, ”CSG SAR instrument design and performance,” Proceedings of EUSAR 2016, 11th European Conference on Synthetic Aperture Radar, Hamburg, Germany, June 6-9, 2016
29) Francesco Spadoni, Antonio Delfino, Francesco Richichi, Roberto Capezzali, Isaia Martinazzo, Francesco Quaranta, Mario Frezzini, “CSG SAR waveform generator and echo digitizer design,” Proceedings of the Advanced RF Sensors and Remote Sensing Instruments &Ka-band Earth Observation Radar Missions, (ARSI'14 & KEO'14), ESA/ESTEC, Noordwijk, The Netherlands, Nov. 4-7, 2014
30) Andrea Gallon, Andrea Francioni, Andrea Gallonalerio Grimani, Fabio Covello,Stefano Serva, “CSG End-to-End System Deployment and Calibration within the Evolved Mission Scenario,” Proceedings of EUSAR 2014 (10th European Conference on Synthetic Aperture Radar), Berlin, Germany, June 3-5, 2014
31) Anna Croce, Marco Cutigni, Danilo Vicari, Oreste Trematerra, Mario Profili, Giovanni Valentini, Mauro Cardone, Efer Motti, ”CSG Ground Segment and ILS&OPS evolution between two generations of systems,” Proceedings of EUSAR 2016, 11th European Conference on Synthetic Aperture Radar, Hamburg, Germany, June 6-9, 2016
32) Pasquale Salemme, Valerio Grimani, Alessandro Cricenti, Ignazio Rana, Stefano Federici, Davide Rizzato, Manfredi Porfilio, Luca Fasano, Stefano Serva, ”CSG mage quality and calibration approach,” Proceedings of EUSAR 2016, 11th European Conference on Synthetic Aperture Radar, Hamburg, Germany, June 6-9, 2016
33) G. F. De Luca, et al., ”COSMO-SkyMed: The F-DUGS integration within the system,” 16th Ka- and Broadband Communications, Navigation and Earth Observation Conference, Milan, Italy, October 20-22, 2010
34) G. F. De Luca, et al., ”Improving the access to COSMO-SkyMed System: Portable User Terminals ready to operate,” 17th Ka and Broadband Communications, Navigation and Earth Observation Conference, Palermo, Italy, October 3-5, 2011
35) ”COSMO-SkyMed Seconda Generazione:System and Products Description,” ASI, 8 February 2021, URL: https://www.asi.it/wp-content/uploads/2021/03/CSG-Mission-and-Products-Description-defpdf.pdf
The information compiled and edited in this article was provided by Herbert J. Kramer from his documentation of: ”Observation of the Earth and Its Environment: Survey of Missions and Sensors” (Springer Verlag) as well as many other sources after the publication of the 4th edition in 2002. - Comments and corrections to this article are always welcome for further updates (eoportal@symbios.space).
Space Segment Launch Mission Status Launch Sensor Complement Ground Segment References Back to top